First published in: American Bee Journal, December 2015
Reevaluating Beebread: Part 3
For Preservation or Digestion?
Randy Oliver
ScientificBeekeeping.com
First published in ABJ Dec 2015
CONTENTS
Anderson’s Investigatory Approach
Previous Research
Identification Of The Microbes Involved (via DNA Sequencing)
Bacterial Abundance During The Fermentation Process
Nurse Bee Feeding Preference By Age Of Beebread
The Pollen To Microbe Biomass Ratio
The Degree Of Digestion Of Hive-Stored Pollen
Dr. Anderson’s Conclusions
Summary
Next
References And Notes
We’ve now identified the bacterial players. So let’s see what Dr. Kirk Anderson’s lab has found to date about why bees turn raw pollen into beebread.
In this article, I’m going to briefly summarize Dr. Anderson’s well-designed investigations [1]. My readers know that I can be critical of sloppy science; conversely, I feel that truly exemplary scientific research should receive kudos. The investigation by Kirk and his associates meets this standard, and is a textbook example of good scientific research and writing. Kirk laid out his investigation methodically, questioning each premise of the “predigestion hypothesis,” and seeing whether there was actual evidence either supporting or refuting the claim. I will quote from Kirk’s papers extensively in italics [2].
Anderson’s Investigatory Approach
Scientists typically specify the hypothesis to be tested and lay out their game plan:
It was hypothesized that co-evolved microbes orchestrate the long-term conversion of stored pollen into a more nutritious food source, a process involving microbial succession, anaerobic breakdown of materials, the release of pollen cell contents and/or predigestion by moulds. Here, we use a multifaceted approach to determine whether hive-stored pollen of honey bees involves significant nutrient conversion or ‘pre-digestion’ by microbes. To this end, we explore [reordered]
- whether the differences in bacterial richness and diversity between newly collected and hive-stored pollen are consistent with a preservation or nutrient conversion environment
- the absolute number of bacteria in stored pollen,
- the association between bacterial abundance and pollen storage time,
- the time period associated with pollen storage prior to ingestion by nurse bees,
- the pollen to microbe biomass ratio, and
- the degree of digestion of hive-stored pollen
Previous Research
Hypothesis to be tested: whether the fermentation of beebread requires inoculation of the pollen with symbiotic “core” gut bacteria via the crop as proposed by Vásquez and Olofsson [3]: “Our research has identified the bacteria involved and revealed that bees, in producing bee bread, add all the beneficial [lactic acid bacteria] to the pollen when they collect it at the site of the flower.”
Vásquez and Olofsson’s early research was very exciting, but they apparently overstated the case in claiming that bees added all the beneficial lactic acid bacteria to pollen, and especially that those bacteria came from the crop. Scientists already knew that pollen, and especially nectar, typically contains lactic acid and other bacteria prior to being visited by bees [4]. And subsequent research by others found that there were very few bacteria in the bee crop [5]. The question then is whether the pollen loads of returning foragers showed signs of being spiked with the core gut bacteria.
What Anderson found: In previous work, we determined that freshly-collected pollen from returning foragers or beebread contains incidental amounts of core hind-gut bacteria, suggesting that this core gut community does not contribute substantially to the conversion or preservation of pollen stores… This new finding contrasts markedly with the previous culture-dependent view…
Note: Dr. Anderson writes in a meticulous and precise scientific manner, appropriate for the journals in which he publishes (I again commend him for his exemplary backing up of each of his claims with accurate citations and supplemental data). It is polite scientific convention to say that a finding “contrasts markedly” with a previous view; a layman might say “man, did those guys get it wrong.”
Identification Of The Microbes Involved (via DNA Sequencing)
Hypotheses to test: if pollen is indeed inoculated by the bees with core gut bacteria in order to ferment it into beebread, then the bacterial species composition in beebread would shift towards core bacteria during the fermentation process.
Anderson found that: “The bacterial communities found in hive-stored pollen did not differ from those of newly collected pollen, but both sample types varied significantly by season.” He found that only a small percentage of the bacteria found in freshly-gathered pollen loads were core hind-gut bacteria (in some cases as little as 3/10ths of a percent). Even more telling was that fermented beebread contained an even lower proportion of core gut bacteria than did newly-collected pollen.
Instead, the most common bacteria found in beebread were strains of Lactobacillus kunkeei. This floral/fruit bacterium thrives in fructose-rich aerobic environments, producing lactic acid as a metabolic byproduct, which has the benefit of making its environment inhospitable to competing yeasts and bacteria. Bees create an ideal environment for L. kunkeei by enriching pollen with fructose-rich nectar–thus its rapid preservation of beebread by the process of acidic “pickling.” L. kunkeei is one of the few bacteria that can survive (perhaps in a dormant state) in honey and beebread, likely quickly rejuvenating when exposed to diluted nectar or honey in the crop.
Kirk and others [6]) have found that the bacterial community in bees may differ a bit from hive to hive, and by season or forage, but there is always a “core” hindgut biota (apparently passed via a fecal/oral route of some sort) [7]. The story is as yet far from complete, but it appears that honey bees have a commensal or mutualistic relationship with these core species, but also utilize free-living flower bacteria, such as L. kunkeei, to their advantage. An interesting recent study by Blum [8], working with the fruit fly Drosophila, points out that a host does not necessarily need to maintain a population of endosymbionts, but may instead continually replenish it via its food:
The Drosophila system may represent an alternative mutualism strategy that we term “quotidian replenishment,” which is intended to indicate the need for daily replenishment to obtain a consistent [bacterial] community in the animal. In this model, the symbiotic community in Drosophila is maintained through frequent ingestion from an external reservoir of bacteria [its food]… Furthermore, our study shows that one member of the microbiome, Lactobacillus plantarum, protects the fly from intestinal pathogens. These results suggest that, although not always present, the microbiota can promote salubrious [9] effects for the host.
It appears that the honey bee may use a combination of vertical transmission (colony to daughter colony) of the core endosymbionts, coupled with the advantageous use of naturally-occurring floral bacteria (similar to the way that humans make sauerkraut or silage). Of interest is that some free-living bacteria can exert salubrious [10] effects on host immunity (which opens the market for bee probiotics) [11].
Bacterial Abundance During The Fermentation Process
Hypothesis tested: if pollen is indeed digested by core bacteria during the fermentation of beebread, then the number of those bacteria would increase during the process.
What Anderson found: that bacterial abundance increased only during the first two days of fermentation, and then decreased thereafter (Fig. 1). This finding does not support the digestion hypothesis, instead indicating that the formation of lactic acid acts to protect the pollen in beebread from microbial degradation.

Figure 1. After a day’s fermentation, the bacterial count of stored pollen increased greatly from that of fresh incoming pollen, and then dropped sharply as the beebread became too acidic for further microbial growth. After Anderson (2014) [[i]].
[i] Kirk shared the raw data so that I could chart it out in a manner more understandable to the layman. See the original paper for his graph, which contains more information.
Nurse Bee Feeding Preference By Age Of Beebread
Hypothesis to be tested: if fermentation improved the nutritional quality of pollen, then it would follow that nurse bees would preferentially consume beebread that had fully fermented.
The Method: Kirk’s team meticulously tracked the daily deposition or consumption of pollen in every cell of an active brood frame from each of 8 colonies over 5 days, repeated with different colonies in March, April, and May.
What Anderson found: The results are shown in Fig. 2. Although these measurements were taken during times of pollen surplus (as indicated by the fact that nearly 90% of the beebread cells were over 96 hrs old), the nurse bees appeared to preferentially consume either fresh incoming or slightly fermented pollen to aged beebread. This observation does not support the hypothesis that fermentation improves pollen nutritional quality.

Figure 2. Measurements were taken of the ages of beebread cells in the combs; the orange columns indicate the average number of cells present for each age class of beebread. The capped lines indicate the proportion of that age class of pollen consumed each day. There was apparently an aversion by bees to consume pollen that had undergone extended fermentation [[i]].
[i] Or perhaps a preference for fresh, or slightly fermented, pollen. The overall expected consumption rate for each age class of beebread predicted to be consumed at random based on its abundance on the frames was 9.3%. Compare that to the measured consumption rates shown. Kirk has shown me additional (as yet unpublished) data gathered this spring that shows that the relative consumption rate continues to drop for beebread aged 6, 7, or more days.
Note: despite the observation that bees clearly prefer fresher beebread to older, due to the overwhelming presence of aged beebread in the combs of the colonies he observed, the majority of total beebread consumed (other than the unmeasured consumption of freshly-gathered pollen) was over 96 hrs old, suggesting perhaps that bees make an effort to “rotate the stores.”
The Pollen To Microbe Biomass Ratio
And now Anderson, in a stroke of brilliance, shot right to the crucial question: are there even enough bacteria present in beebread to exert a digestive effect? Bacteria lack teeth, and thus depend upon the enzymes that they produce in order to digest foodstuffs. Pollen does not make it easy for microbes to get to the good stuff inside the protective outer shell.
A pollen grain is enclosed in a tough coat called the exine. The exine is composed of a highly decay-resistant biopolymer called sporopollenin. Sporopollenin is so resistant to bacterial enzymes that “Pollen walls…often survive intact and recognizable for millions of years in bog and sediment deposits” [14]. The only place for bacteria to gain access to the nutritious innards of the pollen grain is through the (generally) one or more germination pores in the exine (these also serve as routes for water uptake). These pores are protected by a membrane called the intine, composed of cellulose and pectin. It is this layer that bacteria would need to digest their way through.
Kirk recognized that for bacteria to breach the intine of the pollen pores, that there would need to be a lot of them producing enzymes at those surfaces. So are there enough present? As I’m want to say, let’s do the math! In this case, Kirk did it for us.
Hypothesis: that there are enough enzyme-producing bacteria present in beebread relative to the surface area of the pollen grains to digest their way through the pollen shells.
Kirk’s math: He was generous on his estimate of the number of microbes present in mature beebread, quadrupling his actual colony counts to allow for culture bias and fungi, arriving at about 36,900 microbes per gram of pollen. He then counted pollen grains under a scope, estimating about 90 million pollen grains per gram. This works out to about 1 microbe per 2500 pollen grains (Fig. 3). To place this into biological perspective, a single grain of pollen in the bee hindgut is often covered with hundreds or thousands of bacteria (Fig. 4), busily digesting the remnants left over from the digestion that took place in the bee midgut.
As an imaginary visual analogy, I calculated that the proportion of bacteria per surface area of the pollen grains works out to the spatial equivalent of a single BB placed on a football field [15]—hardly enough to produce the enzymes necessary to digest their way through the pollen exine or intine.

Figure 3. A scanning electron micrograph of dried beebread. The pink substance is dried simple sugars, which constitute about 40-50% by weight. Note the distinct lack of bacteria. Electron micrographs courtesy Kirk Anderson.

Figure 4. For comparison, these pollen grains from a nurse bee hindgut are covered with live bacteria (stained purple).
Conclusion: there simply aren’t enough microbes present in beebread to cause any significant degree of digestion of the pollen grain contents. Kirk succinctly concludes that: “Hive-stored pollen lacks the microbial biomass needed to alter pollen nutrition.”
The Degree Of Digestion Of Hive-Stored Pollen
Kirk is hardly the first bee researcher to point out that beebread contains few microbes. Back in 1983 Klungess and Peng [15], using microscopy, found few microorganisms in fresh beebread (but more in dark, old beebread). They concluded that:
The substances, presumably nectar or diluted honey and enzymes from the ventriculus and salivary gland, that bees add to pollen during packing on to the corbiculae [pollen baskets] and into cells, and the bee bread ripening process, do not break down the pollen contents. Nor do the microorganisms associated with fresh and old bee bread appear to cause destruction of pollen intine or the cytoplasm. .. It is, therefore, proposed that the substances added to pollen by bees during storage function as a preservative. We found no visible evidence that these substances pre-digest the pollen so as to make the nutrients more available to bees during subsequent digestion and absorption.
Compare this to Peng’s previous finding that most (but not all) pollen grains are rapidly digested during their passage through the nurse bee midgut, which contains few microbes [16].
Hypothesis: if bacteria indeed digest the pollen grains in beebread, this should be easily observed through microscopy.
Anderson’s finding: “There were no discernible morphological differences between newly collected and hive-stored pollen.”
This should be something that any beekeeper could easily verify for him/herself. So I looked at aged beebread from my own hives under the scope (at 400 and 1000x), and also found very few microorganisms or digested pollen grains (Figs. 5-7).

Figure 5. Pollen grains in aged beebread under light microscopy. Note the relative absence of yeast or bacteria, and the unbreached (not misshapen) exines and intact colored contents of the pollen grains.

Figure 6. Pollen grains from beebread that I diluted with a weak sugar solution and allowed to ferment for 10 days. Note the abundant yeast and bacterial cells in the background (unfortunately there was not enough depth of field to bring both the pollen and microbes simultaneously into focus). Despite being exposed to over a week of vigorous aerobic fermentation, these pollen grains remain intact and undigested. Compare these intact grains to those below.

Figure 7. A sample of digested pollen from the hindgut of a nurse bee. Note how most of the pollen shells are empty (no longer yellow inside) and distorted, indicating digestion of the contents.
Conclusion: the bee midgut can quickly empty pollen grains and digest the contents. But such digestion does not occur when bacteria and yeast ferment pollen into beebread.
Dr. Anderson’s Conclusions
Our combined results do not support the hypothesis that hive-stored pollen of honey bees involves nutrient conversion or predigestion by microbes prior to consumption.
- The bacterial communities found in hive-stored pollen did not differ from those of newly collected pollen, but both sample types varied significantly by season. This result indicates the lack of an emergent ‘core’ bacterial community co-evolved to predigest pollen.
- Relative to other plant material involving microbial digestion or extensive fermentation, hive-stored pollen contains very few microbes.
- The absolute number of bacteria in hive-stored pollen decreases with storage time, indicating that it is not a suitable medium for microbial growth.
- The preferential consumption of freshly collected pollen indicates that bees have not evolved to rely on microbes or other time-related factors for pollen predigestion.
- The microbe to pollen grain ratio is many orders of magnitude removed from that required to alter hive-stored pollen.
- Regardless of sampled season or the taxonomic character of microbial communities, microscopic examination revealed no intermediate stage of pollen digestion in hive-stored pollen.
Based on these collective findings, we suggest that stored pollen is a preservative environment governed largely by nonmicrobial additions of nectar, honey and bee glandular secretions.
Summary
Flowers and pollinators have coevolved over millions of years, and along with them microbes always at the ready to consume them, or perhaps establish symbiotic relationships. Since microbes are invisible to the naked eye, it is difficult for us to grasp their degree of prevalence in the environment, or to understand their contribution to bee nutrition and health.
There may be a million bacteria (along with yeasts and other fungi) per milliliter in nectar when bees first collect it—fresh nectar is often already starting to ferment [18]. Pollen contains somewhat fewer microbes, but is still very biologically active when first gathered. The greatest concentration of microbes in the hive is in the residual undigested material in the bees’ hindguts—that of a single bee may contain hundreds of millions of bacteria [19].
Kirk points out that the fluctuation of pollen availability has likely “selected for the quick turnover of the most readily available pollen nutrients into a ‘nutritional reservoir’ of living tissue (i.e. larva and worker fat bodies). In such a state, nutritional reserves are better protected from microbial digestion, more quickly shared among hive members and easier to digest than hive-stored pollen.”
The above observation reminds us that hungry nurse bees readily consume larvae that they had previously been lavishing with jelly when it was abundant. The larvae thus function as a living protein reserve that through cannibalism can be quickly converted back into jelly or used to replenish fat bodies.
The honey bee, similar to humans, stores surplus food (that can’t be immediately consumed) for later consumption (mainly to survive the winter). Like us, bees have figured out similar ways to prevent that food from being decomposed by microbes. Both nectar and pollen are prepared for prolonged storage by using a combination of high sugar concentration for osmotic preservative effect (similar to making jam), acidification via bacterial lactic acid production (pickling), and the addition of salivary antimicrobial compounds such as glucose oxidase (food preservatives).
Two key bacteria in this preservation process are the acid-resistant and osmotolerant [20] Lactobacillus kunkeei and Parasaccharibacter apium, both of which appear to be instrumental in hive hygiene, food storage, and larval health.
The microbial community in the hive is dynamic and evolving, not only in the gut of every bee during its short life, but also hour by hour during the fermentation of beebread. The microbiomes of the hive rapidly “adapt to changing diets and conditions not only by shifting community membership but also by changing gene content via horizontal gene transfer.” Bacteria, by continually swapping genes, can adapt and evolve at a rate inconceivable to humans.
In the extended flower-pollinator community, pollinators vector microbes (including pathogens) with a remarkably high degree of efficiency. Graystock [21] found surprisingly efficient transmission of pathogens from bee to bee during flower visitations—the same would be expected to apply to hive or gut bacteria. This is due to the fact that the toilet hygiene of flying insects is rudimentary at best, resulting in plenty of fecal bacteria being inadvertently transferred to flower surfaces during pollinator visits (after all, just think about the degree of pollen transfer from flower to flower). And such transmission can be interspecific—any bee, fly, butterfly, beetle, or wasp can carry floral or pathogenic microbes from one flower to another. The total amount of microbial interchange within the entire flower/pollinator environment is mind boggling to consider, and makes for a highly dynamic system of microbial and pathogen interactions that we are only beginning to understand.
Next
The biological and chemical processes that take place during the fermentation of beebread, yeasts and fungi, probiotics, and the Anderson labs’ current research projects.
References and Notes
[1] Published as Anderson, KE, MJ CarrolL, T Sheehan, BM Mott, P Maes and V Corby-Harris (2014) Hive-stored pollen of honey bees: many lines of evidence are consistent with pollen preservation, not nutrient conversion. Molecular Ecology 23: 5904–5917.
[2] Mainly from the above citation, but also from other published and as yet unpublished research. I also took the liberty to edit or reorder the quotations from his papers for readability.
[3] Vásquez A, Olofsson TC (2011) The honey crop – the holy grail when antibiotics fail. Microbiology Today, 38, 226–229.
[4] Fridman S, et al (2012) Bacterial communities in floral nectar. Environ. Microbiol. Rep 4:97–104.
[5] See my previous articles in this series.
[6] Disayathanoowat, T, et al (2012) T-RFLP analysis of bacterial communities in the midguts of Apis mellifera and Apis cerana honey bees in Thailand. Journal of Apicultural Research 51(4): 312-319.
[7] Don’t be grossed out—the same thing happens with humans and many other animals.
[8] Blum JE, et al (2013) Frequent replenishment sustains the beneficial microbiome of Drosophila melanogaster. mBio 4(6):e00860-13. doi: 10.1128/mBio.00860-13
[9] Salubrious–healthful, beneficial, wholesome. Man, I gotta add this descriptive word to my vocabulary!
[10] I couldn’t wait.
[11] Again, I’ve yet to see any hard data that any probiotic currently on the market is of benefit to bees. But I wouldn’t be the least surprised if some were developed in the near future. But don’t just go feeding any untested probiotic—a recent study found that some can seriously harm bees:
Ptaszyńska, AA, et al (2015) Are commercial probiotics and prebiotics effective in the treatment and prevention of honeybee nosemosis C? Parasitol Res DOI 10.1007/s00436-015-4761-z Open access.
[12] Kirk shared the raw data so that I could chart it out in a manner more understandable to the layman. See the original paper for his graph, which contains more information.
[13] Or perhaps a preference for fresh, or slightly fermented, pollen. The overall expected consumption rate for each age class of beebread predicted to be consumed at random based on its abundance on the frames was 9.3%. Compare that to the measured consumption rates shown. Kirk has shown me additional (as yet unpublished) data gathered this spring that shows that the relative consumption rate continues to drop for beebread aged 6, 7, or more days.
Note: despite the observation that bees clearly prefer fresher beebread to older, due to the overwhelming presence of aged beebread in the combs of the colonies he observed, the majority of total beebread consumed (other than the unmeasured consumption of freshly-gathered pollen) was over 96 hrs old, suggesting perhaps that bees make an effort to “rotate the stores.”
[14] Roulston, THI and JH Cane (2000) Pollen nutritional content and digestibility for animals. Plant Systematics 222: 187–209.
[15] By my math.
[16] Klungness L. M., Peng Y.-S. (1983) A scanning electron microscopic study of pollen loads collected and stored by honeybees. J. Apicul. Res. 22: 264-271.
[17] Peng YS, ME Nasr, et al (1985) The digestion of dandelion pollen by adult worker honeybees. Physiol. Entomol. 10: 75-82.
[18] Castillo, C, et al (2012) Seasonal variation in the titers and biosynthesis of the primer pheromone ethyl oleate in honey bees. J Insect Physiol 58(8):1112-21.
[19] Estimate, Kirk Anderson, pers comm.
[20] Osmotolerance is the ability to live in high-sugar environment that would dessicate other microbes.
[21] Graystock P, et al (2015) Parasites in bloom: flowers aid dispersal and transmission of pollinator parasites within and between bee species. Proc. R. Soc. B 282: 20151371. http://dx.doi.org/10.1098/rspb.2015.1371
First published in: American Bee Journal, November 2015
Reevaluating Beebread: Part 2
The Players
Randy Oliver
ScientificBeekeeping.com
First published in ABJ Nov 2015
CONTENTS
Why Do Bees Go To Such Effort To Prepare Pollen In This Manner?
Which Microorganisms Are Involved?
Establishment Of Nest And Gut Microbiota
What About Antibiotics?
Next
Acknowledgements
References And Notes
In the last two decades, the widespread application of genetic and genomic approaches has revealed a bacterial world astonishing in its ubiquity and diversity [1]. It’s become clear that the superorganism that we call the honey bee colony includes a number of symbiotic bacteria. Some appear to be involved in nutrition and immunocompetence, but we are only beginning to understand which players are involved, and what they do.
In the last installment, I introduced Dr. Kirk Anderson. When hired by the Tucson ARS lab (on the downside of the CCD epidemic) he really did his homework, and boned up on everything known about the honey bee microbiome, from which he summarized the then current state of knowledge thoroughly and succinctly in an open-access paper [2], from which I’ll lift a few snips:
The hive of the honey bee may be best characterized as an extended organism that not only houses developing young and nutrient rich food stores, but also serves as a niche for symbiotic microbial communities that aid in nutrition and defend against pathogens. The niche requirements and maintenance of beneficial honey bee symbionts are largely unknown, as are the ways in which such communities contribute to honey bee nutrition, immunity, and overall health.
Kirk pointed out that ants, termites, aphids, and other insects cultivate beneficial bacterial and fungal symbionts, but that:
Given the depth of understanding in the aforementioned systems, it is alarming to consider that virtually nothing is known about the beneficial microbial symbionts of the honey bee, a social insect vital to the worlds food supply. Many thousands of different microbial strains have been cultured from honey bee colonies [he credits Dr. Martha Gilliam], yet we know virtually nothing of honey bee microbial ecology.
Kirk then laid out a vision of a lab devoted to furthering our understanding of how the naturally occurring microbiota of the honey bee colony contribute to bee health and nutrition. Let me be clear that the Anderson Lab is not the only group looking at the bee microbiome—in the U.S. alone, research in this nascent field is also being performed in the labs of Drs. Nancy Moran, Irene Newton, Jay Evans, Gene Robinson, and Quinn McFrederick [3], among others. This is spawning a new generation of bee researchers with proficiency in state-of-the-art technique and analysis.
It’s abundantly clear that we have a great deal yet to learn about the importance (or lack thereof) of the microbiota associated with the honey bee. But for now, let’s return to the subject of this article: the role of the microbes involved in the process of fermenting raw pollen into beebread. Such fermentation could be for either (or both) of two reasons—for simple preservation, or to enhance its nutritional quality. As Kirk explains [4]:
Nutrient conversion is readily distinguished from food preservation (storage). Although both processes have associated microbial communities, the primary function of food preservation is to prevent microbial degradation… preservation environments composed of plant material (e.g. silage) are typically dominated by Lactobacillus spp. and other acid tolerant microbes. Conversely, nutrient conversion involves an extended time component following the collection of plant matter, massive nutrient turnover prior to consumption and a vertically inherited mutualistic microbe or small community of microbes that emerges as the dominant force in the conversion of recalcitrant plant material.
The questions that arise are then:
- Why do bees go to such effort to prepare pollen in this manner?
- Which microorganisms are involved, and do the bees inoculate the beebread with specific symbiotic microbes?
- What chemical and biological processes occur during the fermentation process?
- What are the nurse bees’ consumption preferences?
- Is the fermentation necessary to release the nutrients of the pollen?
- Is the pollen made more nutritious during the fermentation process?
In a relatively short period of intense investigation, Kirk and his associates were able to obtain (at least preliminary) answers to most of the above questions, published in an open access paper [5]. So let’s cover each of the above questions in turn.
Why Do Bees Go To Such Effort To Prepare Pollen In This Manner?
Let’s first consider the dynamics of pollen within the hive. Pollen is the predominant source of protein and other colony nutrition; a continual supply is thus critical if brood is to be reared. Unfortunately, that supply is completely dependent upon an ever changing bloom and capricious weather. When colonies are in their rapid growth phase, or during pollen dearths, there is often little pollen to be seen in the combs. But that doesn’t mean that the colony doesn’t have a reserve stored. So where is it?
In the previous installment I described how the pollen foragers place their loads into cells adjacent to the broodnest, after which mid-aged bees pack it into place for storage. But this then creates the problem of how to protect such a rich larder of stored food (at the warm temperature of the broodnest) from the slew of pollen-feeding insects, mites, fungi, and bacteria eager to consume or decompose it. So what to do?
As Kirk observes, “nutrition stored within the body of an individual is protected by anatomical barriers and active immune physiology.” So nurse and mid-aged bees ravenously consume that freshly-stored pollen, thus protecting it within their bodies (Fig. 1).

Figure 1. Forager bees (sampled from the entrance) tend to have very little pollen in their guts, but “house bees” (nurses and mid-aged bees) tell a different story. I took this sample of 50 bees from an outside frame of the upper hive body in early November (after 2 days of confinement by rain), froze them to kill them, and then crushed them with a roller to squash out their gut contents. Note that the gut of every single bee was full of pollen. In other samples taken during the summer pollen dearth, a small proportion of bees from similar combs lacked pollen in their guts.
A question: The pollen in the above photo was mostly in the hindguts of the bees (post normal digestion). I wonder why they retain the remnants of digested pollen grains for so long, rather than defecating immediately. Could it be that additional nutrients are released or generated by the bacteria that thrive in the rectum?
Nurse and mid-age bees digest that consumed pollen and glandularly convert it into jelly (analogous to mammal milk)—which can be considered as the in-hive “currency” of protein, to be equally shared according to need. Any excess is converted to vitellogenin and stored in the fat bodies of all but the foragers. The colony is thus able to distribute protein reserves throughout the colony population as a whole.
In good times though, foragers bring in more pollen than the nurses can consume, so the colony must preserve it in some additional manner. They do so by fermenting it into beebread, using a process similar to that used for the creation of silage or sauerkraut—by allowing lactic acid bacteria to produce their preservative namesake, thus creating an environment hostile to other microorganisms, allowing for the relatively long term storage of pollen for later consumption.
The question then, is exactly…
Which Microorganisms Are Involved?
First, current day genomic research into the hive microbiome is not something that you do with a microscope. The literature is incredibly jargon-heavy, tedious to read, and consists of monster spreadsheets of numbers meaningless to all but those well versed in this arcane field (I’m definitely not one of them). But there is some common terminology and methodology that I need to briefly explain.
Until recently, scientists’ ability to study the microorganisms in the bee gut was largely limited to culturing them in petri dishes, ala Martha Gilliam’s meticulous research. The problem with that methodology is that many gut bacteria are difficult to culture. It was only with the development of PCR (polymerase chain reaction) to amplify scraps of DNA that we could start to identify organisms by parts of their genome alone. This technology was quickly followed by “metagenomics,” which allowed the identification of unknown and unculturable microorganisms from a sample of biological material. By convention, researchers compare differences in the “spelling” of the 16S ribosomal DNA genes present [6] in order to estimate how many different “species” of bacteria are present. From this analysis, they can then create phylogenetic “trees” of relationships. To their amazement, scientists found that we had missed about 99% of species of bacteria in various environments.
Only recently (2003 [7]) did researchers note that there appeared to be a distinctive gut microbiome in the honey bee, furthered by Diana Cox-Foster’s metagenomic analysis of colonies suffering from CCD [8]. We now know that honey bees tend to harbor a fairly consistent community of symbiotic bacteria [9,10], consisting of what appears to be around eight different “phylotypes” (the separation of bacteria into species is not as clear cut as it is for higher organisms [11]; bacteria identified only by DNA analysis are separated into OTUs (Operational Taxonomic Units, each having a group name such as “Firm 5”). In any phylotype, there may be several clades of OTUs (each OTU presumably being a closely-related species or strain, likely adapted to a slightly different niche, with a different biochemical profile, and thus possibly involved in a different symbiotic relationship, or performing different functions) [12]. As researchers refine their methods, they will surely further split these OTUs into additional named species. Each type of bacteria typically occupies a specific niche in the bee (or hive). Based largely upon an excellent recent review by Dr. Nancy Moran [13] and communication with Kirk Anderson, I created the graphic below to show where they are typically found (Fig. 2).

Figure 2. An adult honey bee may harbor a billion bacteria, with roughly 95% residing in the waste material in the hindgut; few survive in the microbe-hostile crop. Foragers bring environmental bacteria from flowers back to the hive–notably Lactobacillus kunkeei, which then thrives in the fructose-rich nest environment (especially in beebread). Other bacteria are nest symbionts, such as the Alpha 2.2 group found in jelly. And then there are the “core” gut bacteria, exhibiting strict niche fidelity within the bee gut.
Establishment Of Nest And Gut Microbiota
Let me first make clear that the research community is at a very preliminary stage at understanding the establishment and functions of the honey bee microbial community. Bacteria and fungi (as well as pathogens [14]) are brought into the hive with the nectar and pollen. Due to colony hygiene and the unfavorable environments of jelly, beebread, and honey, many species do not survive for long. But some find niches to their liking. And then there are other symbiotic bacteria which may be unique to the hive environment or bee gut—apparently transferred from one generation of bees to the next. And although there are “core” and “typical” communities of bacteria in the bees and the hive, they may vary substantially from colony to colony, race to race, and location to location [15].
Of interest is that Kirk recently found that the newly-emerged bees do not need to be exposed to older bees in order to acquire their inoculum of core bacteria—they apparently ingest it from the combs during their first few hours after emergence [16].
Kirk’s associate Lana Vojvodic tracked the establishment of bacteria in the larval gut [17], and Kirk recently found [18] that the worker gut microbial community structure is quickly established in young bees, with the core bacteria showing up within hours. Then over the next few days a process of succession occurs, with pioneer species establishing first, followed by a climax community that persists for the rest of the bee’s life (despite the huge change in diet as bees progress from nursing to foraging). Another group of researchers [19] recently found that the queen bee microbial community follows a similar course of development–with a huge difference–the queens, which are fed only jelly, develop a different climax community than that of the workers who feed them, consisting predominately (and not surprisingly) of the Alpha 2.1 and Alpha 2.2 bacteria associated with jelly.
The Alpha 1 and 2 phylotypes are as yet poorly understood. Researchers have now figured out how to culture some of these bacteria in the lab, so we may soon learn more about them. It’s likely that the bee and the bacteria have coevolved mutually-beneficial symbioses, presumably with natural selection tweaking the bee hindgut epithelial cells to favor colonization by favored bacteria, which may help with the digestion of honey and pollen, produce important molecules not already present in the food, modulate immunity to pathogens, or aid in the metabolism of plant (or agricultural) toxins.
Biology is always a dynamic work in progress, and there is continual evolutionary adjustment of any system. This appears to be occurring (on the evolutionary time scale) with bees and their symbionts. Symbiotic relationships typically begin as parasitic invasion or opportunistic exploitation of a food source (as in undigested gut contents), which may then evolve into commensalism, and eventually mutualism (see box).
Types of Symbiotic Relationships
A parasitic relationship is one in which one member of the association benefits while the other is harmed.
Commensalism describes a relationship between two living organisms where one benefits and the other is not significantly harmed or helped.
Mutualism or interspecies reciprocal altruism is a relationship between individuals of different species where both individuals benefit. In the case of bees and bacteria, the bees provide a habitat and food source, and in turn the bacteria likely aid in digestion, immunity to pathogens, creation of essential nutrients, and detoxification of plant chemicals or pesticides.
Definitions from Wikipedia
|
In the case of the evolution of the honey bee microbiome, certain bacteria invaded the bee gut and fought to establish a niche. Of course, the bee would try to flush out harmful invaders, but some figured out how to adhere to the gut cells and establish a biofilm [20]. The bee and each species of bacteria then evolutionarily worked out some sort of agreement, presumably in which both species benefitted (mutualism).
The least refined relationships appear to be with the Alpha 2.2 Acetobacteraceae and Lactobacillus kunkeei, which are facultative symbionts—meaning that they can exist in other niches (such as in plant nectar), but some strains also thrive when bees create a hospitable habitat either within their guts or elsewhere in the hive (as in the jelly or beebread). Then there is Frischella perrara, an early invader of the guts of young bees, which causes formation of a scab (see the great photomicrographs at [21]). This bacterium may be transitioning from a parasitic to perhaps a commensal relationship. Finally, there are the highly refined and apparently mutualistic relationships with Snodgrassella and Gilliamella (and of the two species with one another). These species are perhaps only found only in the bee gut, where they form biofilms in the nutrient-rich ileum (where the bee absorbs the digestion products of pollen from the midgut). We do not yet know to what extent the bees depend upon them for health or survival.
One might ask why there are so few groups of bacteria in bees compared to the thousands associated with humans. One researcher suggests that it may be because mammals have a more developed adaptive immune system than do insects (by creating antibodies), which allows for the regulation of more complex relationships [22], or perhaps the abundance of real estate in the mammalian gut simply provides a greater number of distinct neighborhoods for bacterial colonization.
We are only beginning to grasp the complexity of the system; this is where Kirk’s lab is focusing its attention—to try to understand where bacteria and fungi fit into the hive environment as a whole. What I really appreciate about Kirk is that he’s scientifically skeptical (as am I) of nearly everything claimed about honey bees until he’s seen it tested and confirmed. So his lab has gone back to the beginning, assuming nothing, and confirming or refuting several previous “findings” regarding the hive microbiome.
For example, Kirk’s associate Vanessa Corby-Harris continued Vojvodic’s research, and recently named a species of Alpha 2.2 bacteria (Parasaccharibacter apium) which she found in the jelly produced by the hypopharyngeal glands of nurse bees [23]. Of interest is that jelly is clearly strongly antibacterial, yet she found bacteria that not only thrive in jelly, but confer mutualistic benefit to the larvae. Her research suggests that the source of inoculum of symbiotic bacteria to larvae may be the jelly, rather than the contents of the crop.
Practical application (probiotics): to date, there is only suggestive research that the gut symbionts confer appreciable benefit to the bee (this will surely become a hot topic of research). Nevertheless, there are plenty of sellers eager to market “probiotics” to beekeepers. The two predominant bacterial phylotypes in the bee gut are unnamed Lactobacilli, in the same group of bacteria that ferment yogurt, sauerkraut, and pickles. But don’t think that feeding yogurt, pickles, or any off-the-shelf probiotic will help your bees, since each of the many types of Lactobacillus is very specific to a certain niche. There will likely eventually be proven products on the market, but I don’t know of any as of yet.
What About Antibiotics?
Similar as to in your gut, the community of bee gut microbiota are in a constant competitive battle for real estate. If you enjoy street tacos in Mexico as I do, you likely have experienced what happens when a new player enters the neighborhood in your gut. Similarly, the application of antibiotics to your gut (or a hive) can shift the balance by (temporarily) knocking out some susceptible species. As suggested by Dr. Nancy Moran:
It would seem prudent to avoid overuse of antibiotics, as this could have detrimental consequences for colony health, just as chronic use of antibiotics might affect human health by continually perturbing resident gut communities.
But the gut bacteria don’t give up easily, and eventually incorporate genes for resistance to regularly-used antibiotics. This has happened with the U.S. managed bee population, following decades of exposure to oxytetracycline [24].
Does this hurt the bees? Perhaps at first, but I remember that back before varroa, when many of us treated twice a year with oxytet, we sure had healthy hives. A recent experiment by Drs. Eric Mussen and Brian Johnson didn’t find any negative effect on the growth of colonies after feeding a blast of antibiotics [25].
Practical application (antibiotics): there are two extreme views—(1) bees should never be fed antibiotics, and (2) antibiotics should be given prophylactically twice a year to prevent disease. Those promoting View 1 have apparently never had a sick kid whose life was saved by a course of antibiotics; the others are likely wasting money on risk management, chancing contaminating their honey with residues, and breeding antibiotic-resistant bacteria (keep in mind that only when some beekeepers created constant exposure to the antibiotic by using extender patties did we finally see AFB bacteria develop resistance to the treatment).
I suspect that much of the money spent on fumagillin, oxytet, and tylosin is not cost effective, and would better be spent on better nutrition and mite management. On the other hand, when actual monitoring indicates that the presence of a pathogen exceeds a threshold value, treatment can be of great benefit. This was the case in my operation in January, when EFB showed signs of going rampant. We treated all our hives prophylactically for the first time in over a decade, and were pleased with the results. But I have no intention of doing so next season unless clearly called for. The reasons? It’s expensive and time consuming to treat, and I want the antibiotic to still be effective the next time I need it.
Next
In the next installment we’ll delve into the details of what occurs during the fermentation of pollen into beebread.
Acknowledgements
I thank Pete Borst for his generous assistance in literature search, and Dr. Kirk Anderson for the great deal of time spent in discussion and explanation of this research.
References And Notes
[1] McFall-Ngaia, M, et al (2013) Animals in a bacterial world, a new imperative for the life sciences. PNAS 110(9): 3229–3236. http://web.stanford.edu/~fukamit/mcfall-ngai-et-al-2013.pdf This excellent review covers our current state of knowledge of symbiotic bacteria in general (not just for bees).
[2] Anderson, KE, et al (2011) An emerging paradigm of colony health: microbial balance of the honey bee and hive (Apis mellifera). Insect. Soc. 58: 431–444. http://naldc.nal.usda.gov/download/57606/PDF. This is the best open access paper to read for a deeper understanding of the subject, including details of honey bee digestion, state-of-the-art genetic sequencing technology (and discrepancies in analysis), descriptions of the eight “core” bacterial groups, and the microbial niches present in the hive environment. Note that this is a very hot topic these days, with new research coming out monthly.
[3] These researchers communicate informally, as well as by reviewing each others’ work, as explained in a recent blog by Quinn McFredrick: https://melittology.wordpress.com/2012/04/03/bee-microbiome-initiative/
[4] Snipped and paraphrased from Anderson, KE, et al (2014) Hive-stored pollen of honey bees: many lines of evidence are consistent with pollen preservation, not nutrient conversion. Molecular Ecology 23: 5904–5917.
[5] Anderson, KE, et al (2014) ibid.
[6] For you techies: for phylogenetic classification, the 16S ribosomal RNA gene is commonly used because of its ubiquitous presence in all living organisms, its small size, and its alternating structure of highly conserved (slowly evolving) and hypervariable regions. Researchers can analyze either the DNA or the RNA, which can then suggest the functions of each species (metabolism of certain sugars, creation of vitamins, digestion of cellulose, detoxification of plant chemicals, etc.).
[7] Jeyaprakash A, et al (2003) Bacterial diversity in worker adults of Apis mellifera capensis and Apis mellifera scutellata (Insecta: Hymenoptera) assessed using 16S rRNA sequences. J Invertebr Pathol 84:96-103.
[8] Cox-Foster (2007) cited in previous installment of this series.
[9] Martinson VG, et al. (2011) A simple and distinctive microbiota associated with honey bees and bumble bees. Mol Ecol 20:619–628.
[10] Moran NA, et al (2012) Distinctive gut microbiota of honey bees assessed using deep sampling from individual worker bees. PLoS ONE 7(4): e36393. doi:10.1371/journal.pone.0036393
[11] Newton, I (2012) The utility of bacterial nomenclature. http://microdiv.blogspot.com/2012/08/the-utility-of-bacterial-nomenclature.html
[12] Engel, PE, et al (2012) Functional diversity within the simple gut microbiota of the honey bee. PNAS 109(27): 11002–11007.
[13] The best current review of this is by Nancy Moran (2015) Genomics of the honey bee microbiome. Current Opinion in Insect Science 10: 22–28.
[14] Graystock, P, et al (2015) Parasites in bloom: flowers aid dispersal and transmission of pollinator parasites within and between bee species. Proc. R. Soc. B 282 http://dx.doi.org/10.1098/rspb.2015.1371 This exemplary experiment demonstrated how quickly and easily pathogens are transmitted between colonies via flowers.
[15] Disayathanoowat, T, et al (2012) T-RFLP analysis of bacterial communities in the midguts of Apis mellifera and Apis cerana honey bees in Thailand. Journal of Apicultural Research 51(4): 312-319.
Vojvodic S, SM Rehan, KE Anderson (2013) Microbial gut diversity of Africanized and European honey bee larval instars. PLoS ONE 8(8): e72106.
[16] The timing suggests that the inoculum may come from the meconium ingested during cell cleaning (which would imply that those bacteria remain viable over the 12 days post pupation), or from the nectar or honey consumed for the bee’s first meal, since newly-emerged workers typically do not begin consuming beebread until their second day.
[17] Vojvodic (2013) Ibid.
[18] Anderson, K, et al (in press) Ecological succession in the honey bee gut: Colonization by core bacteria during early adult development.
[19] Tarpy, David, Heather Mattila, Irene Newton (2015) Development of the honey bee gut microbiome throughout the queen-rearing process. Appl Environ Microbiol 81:3182–3191.
[20] Stones, DH & A-M Krachler (2015) Fatal attraction: how bacterial adhesins affect host signaling and what we can learn from them. Int J Mol Sci 16(2): 2626–2640 (open access).
[21] Engel, P, et al (2015) The bacterium Frischella perrara causes scab formation in the gut of its honeybee host. MBio doi: 10.1128/mBio.00193-15
[22] McFall-Ngai M (2007) Adaptive immunity: Care for the community. Nature 445(7124):153.
[23] Corby-Harris, V, et al (2014) Origin and effect of Alpha 2.2 Acetobacteraceae in honey bee larvae and description of Parasaccharibacter apium gen. nov., sp. nov. Appl. Environ. Microbiol. 80(24):7460.
[24] Tian, B, et al (Moran lab) (2012) Long-term exposure to antibiotics has caused accumulation of resistance determinants in the gut microbiota of honeybees. http://mbio.asm.org/content/3/6/e00377-12.full
[25] Johnson, BR, W Synka, WC Jasper & E Mussen (2014) Effects of high fructose corn syrup and probiotics on growth rates of newly founded honey bee colonies. Journal of Apicultural Research Volume 53, Issue 1: 165-170.
First published in: American Bee Journal, October 2015
Reevaluating Beebread: Part 1
The “Story”
Randy Oliver
ScientificBeekeeping.com
First published in ABJ Oct 2015
CONTENTS
Introduction
The Heyday Of Bee Research
Pollen And Beebread
The Roots Of A Beguiling Story
Doubting Thomases (Skeptical Questioning)
Enter Dr. Kirk Anderson
Acknowledgements
References and Notes
People feel compelled to come up with explanations for what they observe; and beekeepers are no different. Historically, beekeepers embraced fanciful myths to explain what they saw; frankly, the situation in today’s “age of reason” is often little different. And not even scientists are immune to a beguiling story that appears to elegantly explain some aspect of bee biology.
Introduction
People tend to anthropomorphize the honey bee, meaning that they liken its biology and behavior to that of humans. The fact is, that honey bees are far from human, and see and experience the world very differently than do we. What “makes complete sense” to a human may have absolutely no relevance to the honey bee. Unfortunately, the original sources of some “facts” about the bee come from someone who explained something so compellingly in human terms, that it immediately resonated with beekeepers, who accepted it uncritically and without supporting evidence. We then get “proof by repeated assertion,” with one authority after the next retelling it without question—in bee books, scientific papers, and media articles (it’s the rare reporter these days who actually goes to the trouble to deeply check his facts).
Science, on the other hand, is all about skepticism, requiring impeachable evidence, and continual reevaluation of what we “know.” Those who have been following my writing already know that I’m not afraid to bust some common myths about the honey bee or its situation. So I was surprised by the lack of buzz when a little-known ARS bee researcher recently toppled one of our most cherished tenets. His findings put the lie to a popular myth—that bees need to ferment pollen into beebread in order to digest it.
The Heyday Of Bee Research
There’s been a great deal of scientific interest in honey bees since we began to feel the impact of the evolving varroa/virus complex, the invasion of Nosema ceranae, and changes in agricultural practices; and even more so after Dave Hackenberg brought Colony Collapse Disorder to worldwide attention in 2007 [1](Fig. 1).

Figure 1. Bee research has enjoyed a heyday as beekeeping in the U.S. and Europe began to become more difficult in the early 2000’s. Data from ScienceDirect.
The upside from all this research is that we’ve learned a helluva lot about bee biology in the past decade. In this article I’ll attempt to trace how the enticing story of the need for bees to ferment pollen into beebread grew, and how a team of bright researchers slapped us back to reality.
Pollen And Beebread
Beekeepers have long known how critical an abundant supply of pollen is for good colony performance, either coming in fresh, or stored within the colony [2]. The thing to keep in mind is that the forager bees that gather pollen do not eat it themselves, since when they transition to foraging, they stop producing the proteolytic enzymes necessary to digest it. So the foragers unload the pollen they’ve gathered directly into open cells located at the interface between the brood and stored honey, creating a typical band of beebread (Fig. 2).

Figure 2. Note the band of stored pollen in the interface between the brood and honey. The freshest pollen is bright, whereas older stored pollen has a wet appearance due to the addition of a thin layer of honey.
After a forager places her load of pollen into a cell, mid-age bees then use their heads to pack it firmly into place, pushing out the air as well as adding more nectar, honey, or glandular secretions [3]. Other foragers then place additional loads on top, often resulting in multicolored layers (Fig. 3).

Figure 3. The different colors indicate that each layer of beebread may consist of pollen from a different plant species. Foragers appear to seek out a diversity of pollen sources, which would help the colony to obtain a more nutritionally balanced diet.
Once the cell is about three quarters filled and well packed, the pollen processors then seal it with a layer of honey, under which it ferments into beebread. Humans have long known how to ferment food products in order to preserve them (e.g., sauerkraut, pickles, and cider), or to improve their digestibility (e.g., yogurt and ripe cheese) or flavor (e.g., miso and vinegar), or their chemistry (e.g., beer). So of course we resonated with the concept that our beloved bees also practiced zymurgy [4]. What a great story, huh? And like many great stories, it got better with each retelling.
The Roots Of A Beguiling Story
I spent a bit of time in trying to trace the development of the beebread story. Back in 1957, popular columnist Len Foote, in an article in ABJ entitled “Possible use of microorganisms in synthetic bee bread production” [5] wrote:
The need for effective and economical substitutes for pollen and bee bread is becoming increasingly more apparent and urgent in the field of beekeeping…Every effort is being made at the present time to more accurately determine the nature and composition of both pollen and bee bread in order to produce effective and economical substitutes…we now have formulas that show promise of being effective substitutes for fresh pollen; but, as yet, there is no formula that promises to take the place of bee bread in the diet of the colony.
After reviewing the research to date, Foote concluded that:
It is quite obvious that bee bread is more than stored pollen.
Dr. Mykola Haydak seconded that opinion the next year in ABJ [6], suggesting that pollen subs [7] might be improved by fermenting them in a similar manner. Oddly though, in the 1963 edition of The Hive and the Honey Bee, Haydak only mentioned that “Lactic acid produced by bacteria preserves beebread from spoilage.”
The heyday of bee nutrition occurred between 1969 until the invasion of varroa around 1990, when research attention turned to the mite. It seems from reading the literature that there was a bit of disagreement between legendary bee nutritionist Dr. Elton Herbert Jr. at the Beltsville lab (working on artificial diets), and Dr. Martha Gilliam at Tucson (who saw things through the eyes of a microbiologist) [8]. Researchers at the Tucson lab found intriguing suggestions that pollen nutritional value improved after fermentation [9], but could only state that:
Published reports are inconclusive and contradictory concerning the relative composition and nutritive value of pollen before and after it is stored by bees… The fact is that chemical and biochemical changes occurring in pollen after bees have stored it in comb cells are not clearly understood, though numerous investigators have offered suggestions as to possible mechanisms involved. Such information is an essential pre-requisite to the development of an adequate artificial protein diet for honey bees and to understand the complexities of the nutritional requirements.
There were plenty of suggestive reasons for Gilliam to believe that bees were using her beloved microbes to convert raw pollen into a more nutritional food. Herbert was more circumspect, and when his findings were summarized posthumously in the 1992 edition of The Hive and the Honey Bee he could only state that:
Stored pollen undergoes a number of biochemical changes which may be responsible for increased stabilization of the product or may lead to chemical changes that increase the digestibility and nutritive value to bees.
Gilliam responded in 1997 in her review [10]:
The fermentation and chemical changes of pollen stored in comb cells by honey bees may be processes similar to those that occur in green plant food materials that are ensiled and in foods of plant origin that are fermented to prolong shelf-life and to improve palatability, digestibility, and nutritional value.
She concluded that the team’s research indicated:
the superiority of the nutritive value and availability of amino acids in the protein of bee bread compared to corbicular pollen…Thus, we hypothesized that an association between Bacillus spp. and some bees may have evolved in which female bees inoculate food sources with these bacteria whose chemical products contribute to the pre-digestion, conversion, enhancement, and/or preservation of food that is stored in the nest.
By the early 2000’s, we were really starting to struggle with colony health issues, so the Tucson ARS lab again began to focus upon bee nutrition, with Dr. Gordon Wardell working to develop an artificial diet [11]. Then came CCD, and beekeepers became aware of the new field of metagenomics [12]. Scientific technology had by this time advanced beyond the petri dish culture techniques that limited Martha Gilliam; researchers could now use a new generation of fancy scientific equipment and high-speed computers in order to screen biological samples for the presence of previously unidentified and unculturable microorganisms. We soon discovered that biological systems (including both the human gut and the honey bee colony) were more diverse at the micro scale than we had ever imagined.
Even more so, it was discovered that the symbiotic microbes in our guts were essential to our digestion, immune function, and the production of critical nutrients (Fig. 4). In 2008 the National Institutes of Health initiated the Human Microbiome Project and the microbiome became a hot topic in both science and the health food market (meaning that it would get a lot of chatter on the internet).
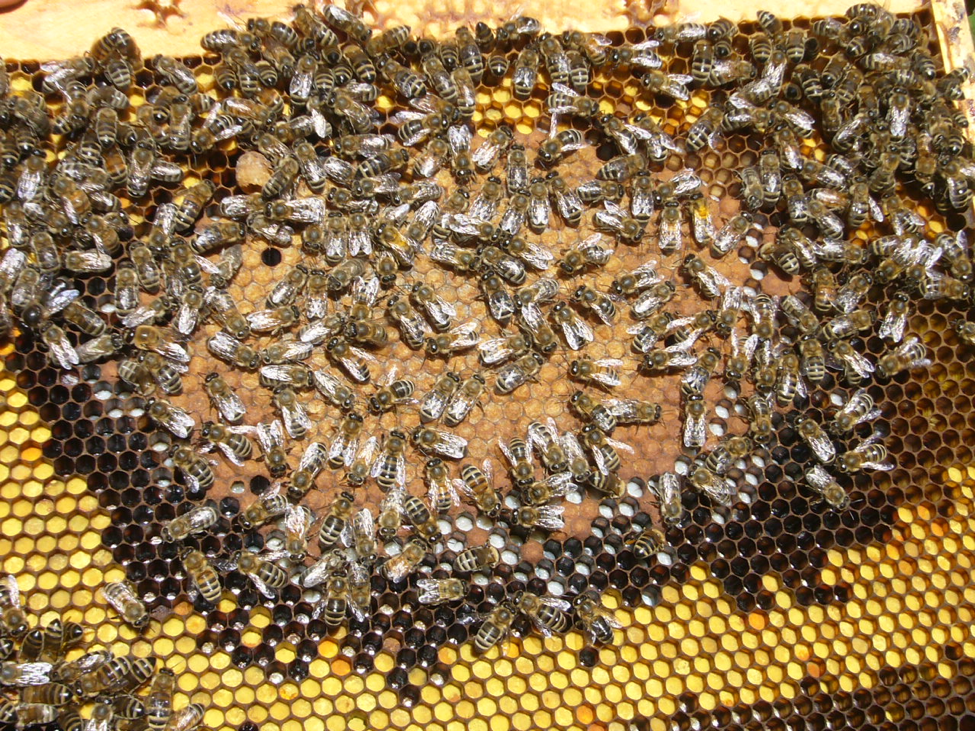
Figure 4. This colony in May had a surfeit of pollen, much of which will soon be glazed with honey and converted into beebread for later consumption. Just how important is such microbial fermentation to the nutritional utilization of the pollen by bees? What is the structure of the microbial community involved? And is it necessary for bees to inoculate the pollen with symbiotic bacteria?
Google Trends shows that interest in “probiotics” took off at that time. Beekeeper Kevin Jester developed a fermented pollen sub. And the Tucson lab, under the direction of Dr. Gloria DeGrandi-Hoffman, explored the potential disruption of the microbial community by fungicides, miticides, antibiotics, or high fructose corn syrup [13]. Dr. DeGrandi-Hoffman pointed out at the national conventions that they were unable to maintain colonies in flight cages on the lab’s artificial diet alone, but could restore them to health if they gave them a bit of natural beebread—a sure indication of the importance of the microbes in beebread.
The beekeeping community, struggling with high rates of colony losses, grasped for reasons. Could we have somehow screwed up the hive microbial community? And as the conventional wisdom grew that the fermentation of beebread was critical for its proper utilization by bees, the timing was ripe for two Swedish researchers to publish new findings [14] claiming that bees inoculated pollen with specific coevolved lactic acid bacteria from their crops in order to start the fermentation process. They of course wasted little time in commercializing a product [15], and briefly collaborated with the Tucson Lab.
The pieces of the puzzle were now all coming together into one alluring story. Dr. Gloria DeGrandi-Hoffman summarized the current state of knowledge in three articles in ABJ in 2009 [16], revving up the engines for revisiting an area of research that had been largely ignored for 20 years. She teased our interest by explaining that:
The processing of pollen into beebread involves a progression of microbes to establish an environment for the fermentation and pre-digestion of pollen, along with the addition of nutrients from the microbes that are essential for optimum honey bee health.
We had now entered into a New Age of holistic beekeeping, in which we were considering the effects of antibiotics, pesticides, miticides, sugar syrups, and pollen subs upon the in-hive microbes. With the new technologies of metagenomics, we now had the chance to finally truly understand the importance of the microbial community in colony health.
Doubting Thomases (Skeptical Questioning)
As a biologist and beekeeper, I was so enthralled by the story of the pre-digestion of pollen by microbes that I bought right into the notion, despite that fact that it was based solely upon suggestive findings and speculation. How easy it was to overlook the fact that the story had a few obvious holes in it.
As pointed out by “Oldtimer” on Beesource [17], any beekeeper can confirm that colonies grow just fine when they’re consuming raw pollen as fast as it comes in, with no time for fermentation. And colonies grow quite well on patties of raw pollen that has not been made into beebread [18]. Somehow, the beebread story must account for such discrepancies.
Earlier Beltsville and Tucson researchers had been unable to demonstrate that fermentation actually improved the nutritional value of pollen [19]. And Fernandes-Da-Silva [20], studying the beebread of stingless bees, found that “the storage of pollen seems to be of no importance in changing the nutritive value.”
Then Dr. Nancy Moran lab’s findings [21] did not support the “symbiotic crop bacteria inoculation” hypothesis of the Swedish researchers:
Even though the crop often contains nutrient-rich nectar that could be used as an energy source for microbes, it contains very few bacteria. The frequent filling and emptying of the crop as nectar is collected and transferred to the hive for honey production could perturb the microbial community and prevent bacterial colonization.
I’ve illustrated the Moran lab’s findings below (Fig. 5).

Figure 5. The lack of bacteria in the crop simply does not support the hypothesis that the inoculation of pollen with bacteria from the crop is essential for the fermentation of beebread—there simply aren’t enough bacteria in the crop. Data after Martinson [[i]], drawing from Snodgras [[ii]].
[i] Martinson (2012) ibid.
[ii] Snodgras, RE (1910) The Anatomy of the Honey Bee. USDA Technical Series, No. 18, Government Printing Office.
Enter Dr. Kirk Anderson
In 2009, the Tucson lab created a position for a “microbial ecologist,” filling it with the top-notch and academically aggressive scientist Dr. Kirk Anderson (Fig. 6), whose previous experience dealt largely with the microbiome of ants. At first, Kirk bought right into the beebread story, and wrote about it elegantly [24].

Figure 6. Dr. Kirk Anderson taking pH readings of beebread with a “pH Spear”– a food industry instrument (fermentation rapidly results in increased acidity of the pollen). When I questioned Kirk about his sparkling white suit, he said that unlike beekeepers such as myself, microbiologists actually wash their suits regularly. Photo by Patrick Maes.
Realizing that he lacked experience with bees, and needing advice in establishing a migratory operation for research purposes, Kirk contacted me, and we’ve since become collaborators and friends, often communicating daily about bee research.
Practical application: I find that the bee research community often benefits from bringing in experts from outside the field. These researchers may then see things through different eyes. I’m happy to say that the Tucson lab now has one of the best and brightest working for us. Kirk is approachable, goes right for the heart of any scientific question, and isn’t afraid to challenge the established conventional wisdom.
Next month I’ll cover what Kirk discovered when he applied his scientific expertise to deeply investigate the microbiota of the hive and its involvement in the fermentation of beebread.
Acknowledgements
Thanks to Pete Borst for his help in research, to Dr. Gerry Loper, and to the ARS for hiring Kirk Anderson.
References and Notes
[1] Another example of a commonly repeated misconception. We beekeepers in California started experiencing CCD in 2004 (this is why the price for almond pollination suddenly spiked); and when I asked Dave whether his bees may have “caught it” in almonds at that time, he told me that he first saw it in his hives in 2003.
[2] As evidenced by Dr. C.L. Farrar’s excellent summary for optimal colony management: Farrar, CL (1968) Productive Management of Honey-Bee Colonies
[3] The process of pollen collection, unloading, and packing is meticulously described by Dr. Mykola Haydak in the 1963 revision of The Hive and the Honey Bee, Dadant & Sons.
[4] Zymurgy: the area of applied science related to fermented foods and beverages.
[5] Foote, HL (1957) Possible use of microorganisms in synthetic bee bread production. Amer. Bee J.97:476-478.
[6] Haydak, MH (1958) Pollen – Pollen Substitutes – Beebread. Amer. Bee J. 98: 145-146.
[7] I’ll use this generic term for both pollen supplement and substitute
[8] A list of Gilliam’s publications can be found at http://www.beeuntoothers.com/Gilliam/Gilliam%20Book%202/TOC.pdf
[9] Loper, GM, et al (1980) Biochemistry and microbiology of bee-collected lmond (Prunus dulcis) pollen and bee bread. I.‒ Fatty acids, sterols, vitamins and minerals. Apidologie 11(1): 63-73. Open access.
Standifer, LN, et al (1980) Biochemistry and microbiology of pollen collected by honey bees (Apis mellifera L.) from almond, Prunus dulcis. II: Protein, amino acids and enzymes. Apidologie 11 (2): 163-171. Open access.
[10] Gilliam, M (1997) Identification and roles of non-pathogenic microflora associated with honey bees. FEMS Microbiology Letters 155(1): 1-10. Open access.
[11] Eventually marketed as Megabee http://agresearchmag.ars.usda.gov/2003/mar/bees/
[12] With the publication of Cox-Foster, D, et al (2007) A metagenomic survey of microbes in honey bee colony collapse disorder. Science 318(5848): 283-287.
Metagenomics for bees are nicely described by DeGrandi-Hoffman, G, et al (2012) Honey bee health: the potential role of microbes, in D Sammataro and J Yoder, eds. (2012) Honey Bee Colony Health : Challenges and Sustainable Solutions. CRC Press.
[13] Yoder, J.A., Condon, M.R., Heydinger, D.J., Hedges, B.Z., Sammataro, D., Finley-Short, J.V., Hoffman, G.D., Olson, E. 2012. Fungicides reduce symbiotic fungi in bee bread and the beneficial fungi in colonies. In: Honey Bee Colony Health, ibid, p. 193-214.
[14] Olofsson, TC & A Vásquez (2008) Detection and identification of a novel lactic acid bacterial flora within the honey stomach of the honey bee Apis mellifera. Current Microbiology 57(4): 356-363.
Vásquez, A &TC Olofsson (2009) The lactic acid bacteria involved in the production of bee pollen and bee bread. Journal of Apicultural Research and Bee World 48(3): 189-195.
[15] https://www.youtube.com/watch?v=dQYY1WUyVD4
[16] Degrandi-Hoffman, G, et al (2009) The importance of microbes in nutrition and health of honey bee colonies (published in 3 parts). American bee Journal 149(6-8).
[17] Discussion introduced by Oldtimer on Beesource thread “Microbial Activity and Pollen Myth.”
Perhaps one of the easiest, if non scientific ways we can observe that bees use pollen that has not been stored and fermented, and any beekeeper can do this, is observing a newly hived swarm. The hive can be opened a few days after being hived and will have brand new comb with eggs and new larvae. The larvae are sitting in white royal jelly. This is despite that the hive may have virtually no pollen in storage, and if there is any pollen in cells it has been there such a brief time it is not even patted down.
To my non scientific head it is clear bees can create bee larvae food from perfectly fresh pollen.
[18] https://scientificbeekeeping.com/a-comparative-test-of-the-pollen-sub/
[19] Herbert, EW & H Shimanuki (1978) Chemical composition and nutritive value of bee-collected and bee-stored pollen. Apidologie 9: 33–40.
Loper (1980) Op cit.
[20] Fernandes-Da-Silva, PG & JE Serrão (2000) Nutritive value and apparent digestibility of bee-collected and bee-stored pollen in the stingless bee, Scaptotrigona postica Latr. (Hymenoptera, Apidae, Meliponini) Apidologie 31: 39–45.
[21] Martinson VG, Moy J, Moran NA (2012) Establishment of characteristic gut bacteria during development of the honey bee worker. Appl Environ Microbiol 78: 2830–2840.
[22] Martinson (2012) ibid.
[23] Snodgras, RE (1910) The Anatomy of the Honey Bee. USDA Technical Series, No. 18, Government Printing Office.
[24] Anderson, KE, et al (2011) An emerging paradigm of colony health: microbial balance of the honey bee and hive (Apis mellifera). Insect. Soc. 58: 431–444.