DRAFT IN PROGRESS updated 24 June 2023
Suggested Text for an Amendment to FIFRA
I have zero legal background, but suggest that we pitch a short and sweet amendment to be placed in the Farm Bill. There is already a section in FIFRA 40 CFR 152.25 regarding exemptions.
§ 152.25 Exemptions for pesticides of a character not requiring FIFRA regulation.
Congress could simply add after exemption (f) Minimum risk pesticides, a brief exemption (g) to exempt the own use of generic natural products by beekeepers. Suggested text:
*********************************************************************************************************************************
A BILL
To amend the Federal Insecticide, Fungicide, and Rodenticide Act to provide an exemption for pesticidal use of specified generic natural substances by beekeepers, limited to application to their own hives.
Be it enacted by the Senate and House of Representatives of the United States of America in Congress
SECTION 1. SHORT TITLE. This Act may be cited as the ‘‘Beekeeper Own Use Exemption of 2023’’.
SEC. 2. EXCLUSION FROM REGULATION UNDER FIFRA.
IN GENERAL. The Federal Insecticide, Fungicide, and Rodenticide Act (7 U.S.C. 136 et seq.) is amended by inserting after section 152.25 Exemptions for pesticides of a character not requiring FIFRA regulation.
‘‘(g) EXEMPTION FOR OWN USE BY BEEKEEPERS OF GENERIC NATURAL SUBSTANCES
‘‘Since the use of generic oxalic acid, formic acid, thymol, or food-grade plant oils applied to bee hives poses no unreasonable risk to man or the environment, the use of these substances for parasite control in one’s own bee hives shall not be subject to regulation under this Act. This exemption is limited to the preparation and application of these generic products by a person for their own use, and does not affect the requirement for registration of products to be advertised or sold for pesticidal purposes.”
*********************************************************************************************************************************
Suggested Pitch to Legislators
This is a case of unreasonable regulation, rather than a question of “unreasonable risk.” The use of commonly-available natural plant products by beekeepers to control the varroa mite should be exempt from regulation by the Administrator of FIFRA.
What our regulators should keep in mind is that people only respect laws that they feel are reasonable. Unreasonable laws tend to be ignored and not enforced. The unfortunate fact is that the entire beekeeping industry has rationalized using unapproved methods to control varroa. This amendment would help to bring beekeepers into compliance with the law, and clarify the law for those responsible for enforcement.
FIFRA’s mandate is that the application of any pesticide should result in “no unreasonable risk to man or the environment.” It’s obvious to any beekeeper that using oxalic acid, formic acid, or thymol in their hive would in no way create either of those risks. Our regulators need to understand that for us to respect them, EPA’s regulations must make “common sense.”
The advantages of this Amendment are:
- This would be a minor amendment to FIFRA included in the Farm Bill, simply adding an additional paragraph to the list of existing exemptions.
- It would apply solely to natural, off-the-shelf biodegradable substances, not to synthetic chemicals.
- Beekeepers would be exempted from regulation soley for the use of (as opposed to the sale of) these specific natural substances.
- It would be limited solely to applications to bee hives by the beekeepers themselves.
- Any preparations of the generic substances could only be made by the beekeeper for their own use.
- EPA would continue to regulate the sale of any products sold for their pesticidal effects against varroa.
- No prepared products could be advertised or sold for pesticidal purposes unless they were registered with the EPA.
- It would be up to the beekeeper to decide how to choose and use each substance or product (in rotation), following guidance by USDA’s Office of Pest Management Policy or by state agricultural extension.
This amendment is along the line of the exemptions for Minimal Risk pesticides and Minor Use Crops (bee hives), and simply bypasses the slow-movng EPA (which focuses on registration and sale of pesticides) by instead adding a tiny amendment to FIFRA (as to the own use of these natural substances by beekeepers) in the upcoming Farm Bill. As far as use in bee hives, these natural substances should all be on EPA’s Minimal Risk list (as far as use in bee hives) anyway, but EPA moves at glacial pace, and has only added a single substance to the list since its writing in 1996.
The EPA’s strict adherence to the letter of the law regarding FIFRA, and to protect themselves from liability or lawsuits, may put them at odds with common sense and beekeeper practical experience. The active ingredients of the organic acids oxalic or formic, or the plant extract thymol, due to their rapid biodegradation, when applied to beehives pose absolutely no unreasonable risk to the environment, and since they all have tolerance exemptions in honey, no risk to the consumer, and with reasonable precaution, no more risk to the applicator than do commonly-used oven or toilet cleaners, bleaches, swimming pool treatments, etc.
This is not about the registration or sale of these substances as pesticides, but is only with regard to the own use of off-the-shelf generic natural substances by beekeepers. Beekeepers already commonly use generic oxalic and formic acids, thymol, or food-grade plant oils in their hives to control varroa mites — this amendment would simply bring them into compliance with the law.
The restriction against selling is a key point that differentiates this bill from a Minimal Risk Exemption, since (1) EPA would continue to regulate these substances in formulated products for sale and (2) current registrants of formulated oxalic, formic, or thymol products would not have wasted money in getting their products registered (so there may be less pushback by them against this, since it still leaves the door open for companies to develop, register, and sell formulated products for beekeepers who would rather purchase tested ready-to-use treatments). It would also promote experimentation and development of new application methods that could then be submitted for regulatory approval).
Beekeepers themselves are in the best position to balance the chemical risks to the applicator involved in using oxalic or formic acids or thymol, versus the EPA requirement to wear bulky, heat-trapping protective gear in hot weather, and the dangerous labor involved in unstacking and restacking honey supers to apply a treatment ― based upon our practical field experience, EPA’s label requirements are ridiculously excessive. Unlike as with the application of neurotoxic synthetics, so long as an applicator wears safely glasses when handling acids, there is little risk of long-term health issues involved in the handling of these active ingredients. Yes, some are acids, but even in risk-averse Europe beekeepers are allowed to handle liquid formic acid (not to mention that in this country aerosol cans of concentrated lye oven cleaner and acid toilet bowl cleaners are routinely used in households ).
There is no need to mention preparation methods — this exemption has nothing to do with how a beekeeper applies a substance.
Here’s the problem: it is perfectly legal for a beekeeper to put generic oxalic or formic acid, thymol, or plant oils into their hives for the purpose of colony health, bee repellency, or the cleaning of frames or combs. Neither the EPA or FDA are concerned about risk to the environment nor to the honey consumer. But if in their mind the beekeeper is using them for pesticidal purposes, they would be breaking the law. Our predicament is that this creates a cloudy situation for beekeepers and those responsible for the enforcement of FIFRA.
New Zealand’s Ministry for Primary Industries, understanding this predicament, and realizing that (1) beekeepers needed help (and would likely help themselves anyway), and (2) that these generic natural substances applied to bee hives posed no risk to man or the environment, wisely granted beekeepers an Own Use Exemption [[i]] for “compounding of substances for their own use.” To wit: “(1) The exemption applies to a substance or compound prepared by a person for use on animals or plants that they own, or on any land, place or water that they own or occupy. (2) In a beekeeping context, the ‘own use’ exemption is commonly used when a beekeeper prepares and applies preparations containing generic substances, such as oxalic acid or formic acid, to their own hives for control of Varroa mites.” The preparations can only be made by the beekeeper for their own use, and cannot be advertised or sold.
The logic for an exemption comes from the text of FIFRA itself:
7 U.S. Code § 136a – Registration of pesticides
(a)Requirement of registration
Except as provided by this subchapter, no person in any State may distribute or sell to any person any pesticide that is not registered under this subchapter. To the extent necessary to prevent unreasonable adverse effects on the environment, the Administrator may by regulation limit the distribution, sale, or use in any State of any pesticide that is not registered under this subchapter and that is not the subject of an experimental use permit under section 136c of this title or an emergency exemption under section 136p of this title.
I boldfaced the key words ― the Administrator may limit the use of a pesticide only if it causes unreasonable adverse effects. Since application of the generic forms of these natural generic substances within the confines of bee hives would clearly not result in any unreasonable effects on the environment, it is not necessary for the Administrator to regulate their use (as opposed to registration or sale of formulated products), and such use should be exempt from regulation under FIFRA. We’d simply be asking for our legislators to codify that fact, not with an emergency exemption, but rather a specific exemption amendment to section 136a.
Additional Material
Pesticide sale or use under FIFRA
7 U.S. Code § 136a – Registration of pesticides
(a)Requirement of registration
Except as provided by this subchapter, no person in any State may distribute or sell to any person any pesticide that is not registered under this subchapter. To the extent necessary to prevent unreasonable adverse effects on the environment, the Administrator may by regulation limit the distribution, sale, or use in any State of any pesticide that is not registered under this subchapter and that is not the subject of an experimental use permit under section 136c of this title or an emergency exemption under section 136p of this title.
UNREASONABLE ADVERSE EFFECTS ON THE ENVIRONMENT.—The term ‘‘unreasonable adverse effects on the environment’’ means (1) any unreasonable risk to man or the environment, taking into account the economic, social, and environmental costs and benefits of the use of any pesticide, or (2) a human dietary risk from residues that result from a use of a pesticide.
The applicable sections of FIFRA are where it defines a “pesticide” as “any substance or mixture of substances intended for preventing, destroying, repelling, or mitigating any pest” (§ 152.3 Definitions). However, FIFRA does allow for certain products to be exempted from registration under 40 CFR §§ 152.25. And FIFRA does recognize beekeepers applying products within their hives as a special case in 40 CFR 152.8(b) A product intended to force bees from hives for the collection of honey crops.
FIFRA also exempts from registration Minimum Risk Pesticides, including thyme oil and a number of acids. Unfortunately, EPA has not yet added generic thymol, or formic or oxalic acids to the List, despite them all being naturally-occurring chemicals commonly produced by plants or animals, all having tolerance exemptions in honey, and exhibiting absolutely minimal risk to man or the environment. It’s pretty obvious that applying these chemicals to bee hives at a dose that does not kill the bees is not going to result in harm to the environment.
Rather than petitioning the EPA to go through the tedious process of adding them to the Minimal Risk list (which would allow pesticidal use of these ingredients by anyone), it would be more straightforward to add a specific §152.25 exemption limited to application of these generic ingredients to within the confines of bee hives, limiting them to a beekeeper’s own use alone, and not allowing sale of any prepared products unless registered by the EPA, or eventually added to the Minimal Risk List.
The example of New Zealand
New Zealand’s Ministry for Primary Industries realized that their beekeepers were already using generic thymol, or formic or oxalic acid in their hives. Since such use obviously constituted no risk to the public or the environment, and having no desire for all beekeepers to be pesticide scofflaws, granted them an exemption when a beekeeper prepares and applies preparations containing generic natural substances, such as thymol, oxalic acid or formic acid, to their own hives for control of varroa mites.
The exemption applies to a substance or compound made from these chemicals, prepared by the beekeeper for use only in hives that they own. The exemption does not apply to registered varroa-control products, nor does it allow the sale of formulated unregistered products made from these generic ingredients.
The beauty of New Zealand’s solution is that Registrants are still free to develop, register, advertise, and market varroa control formulated products for sale (there are several already registered and sold in the country). This exemption places full responsibility for safe preparation and application of these generic products upon the beekeeper themselves, releasing the Ministry from liability.
New Zealand’s own use exemption

Citations
[i] https://www.mpi.govt.nz/dmsdocument/37901-Advertising-and-own-use-guidance-for-compounds-for-management-of-disease-in-beehives-Guidance
Contents
Introduction
Was Another Field Study Justified?
Background Research
The Biology Behind Mite Transfer Between Hives
How Much Mite Immigration Actually Occurs?
How Do the Mites Get Transferred?
How Important is the Drifting of Drones?
How Much Drift is There of Worker Bees?
How Important are the Guard Bees?
Do Mites and Viruses Cause Increased Drifting?
Robbing vs. “Ordinary” Drifting
How Far Can Mites Hitchike a Ride?
Next Month
Acknowlegements
Citations and Notes
A Study on Bee Drift and Mite Immigration
Part 1
Randy Oliver
ScientificBeekeeping.com
First Published in ABJ in February 2023
Many beekeepers complain about sudden late-summer spikes in their varroa counts, blaming them on mites immigrating into their hives from other colonies in the neighborhood. When my sons and I suspected that this was taking place in some our own yards, I decided to run a field study to find out exactly what was happening.
INTRODUCTION
It requires human transport to move the varroa mite across geographical barriers, but to the dismay of beekeepers, once introduced into a country, the mite can spread rapidly to all colonies in the region. This invasiveness is largely due to the natural drift of bees, which also results in the carrying of mites from hive to hive. I wrote about what was then known about mite drift back in the spring of 2018, and suggest that if you’re interested in this subject that you first review my two articles on the subject [[1]].
SO WAS ANOTHER FIELD STUDY ON MITE DRIFT JUSTIFIED?
To answer that question, let’s take a look at data from Ritter in Germany, taken back in 1987 [[2]]. The paper was in German, but worth translating. Ritter set up two separate apiaries of 16 mite-free colonies — one in the foothills of the Black Forest in an area with low colony density and the other in the Rhine plain with numerous heavily infested colonies in the environment, and then tracked the infestation rates of the bees and brood in the experimental hives over time. His results confirmed that having heavily-infested colonies in the neighborhood can make a heckuva difference (Figure 1).
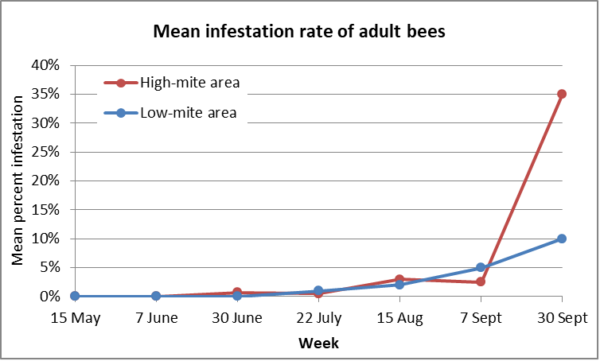
Fig. 1. Of interest is how long it took for the effect to show in the mite washes. At an infestation rate of 35%, that would be 110 mites in a wash of a half cup of bees! Side note: Back then the recommendation in Germany was to use gasoline for performing mite washes. I assume that they were careful with their smokers!
But was the above sudden spike in the infestation rate due to a sudden influx of mites in September? Take a look at Figure 2.
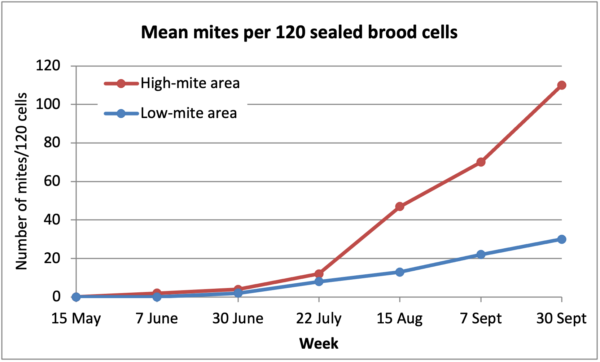
Fig. 2. It appears that the impact of mite immigration actually started to take place long before September. The apparent “sudden” spike as indicated by mite washes may have actually been due to an exponential increase in mite emergence in September, into a decreasing number of adult bees in the hives. This may explain why even beekeepers who are monitoring via mite washes may still get surprised!
Practical application: Ritter’s field data certainly suggests that mite immigration from other colonies in the neighborhood can affect the rate of mite buildup in one’s own hives. So I wanted to dive deeper.
In order to better understand this phenomenon, I modified my mite model [[3]] to run some simulations (after first confirming that the model could produce results in the ballpark of Ritter’s data). Let’s first take a look at the estimations of details of varroa buildup over time in an untreated colony headed towards collapse in October (Figure 3).

Fig. 3. Allow me to use the simulation above to introduce you to the concept of the “Mite Factory.” I added extra plots for daily amount of mite increase, as well as for the estimated amount of mite exodus on outgoing bees. Read below for explanations.
By the time an untreated, nonresistant Mite Factory about to collapse:
- It will typically contain around 15,000 mites in total.
- The above number will be increasing at a net increase of about 150 mites per day.
- And due to the short lifespan of summer bees, above that number, there will likely be over 150 mites hitching a final ride out of the colony each day [[4]].
Unfortunately, there are too many variables for the model to predict the number of those exiting mites lucky enough to be carried to another hive. With the above figures in mind, you may be wondering:
Practical question: What impact might a Varroa Factory in the neighborhood have upon my well-managed (treated) hives?
To answer that question, let’s run the same simulation, but this time with a couple of efficacious treatments (Figure 4).

Fig. 4. Same simulation, but this time treated in April and August, resulting in a far lower mite level in mid-September (around 35). This now gives us two baseline numbers (15,000 and 35) to play with.
Now imagine a neighborhood in which one out of every ten hives is untreated, while the other nine colonies are treated (each containing an average of only 35 mites, as in the second simulation). Let’s see what happens if an arbitrary 10% of the 15,000 mites in the untreated colony somehow manage to evenly “diffuse” (via bee drift) into the nine other treated hives (an average of 165 mites per hive)(Figure 5).

Fig. 5. A single “Mite Factory” has the potential to seriously reinfest all the other hives in the neighborhood after their mid-August treatment (in reality, the distribution of mites would of course be uneven, with some colonies receiving more mites than others). Imagine the effect if your apiary was surrounded by a number of “mite factories”!
OK, so mite immigration could clearly have a noticeable impact if you already had your mites well under control, but what if you were running a little behind the curve? (Figure 6).
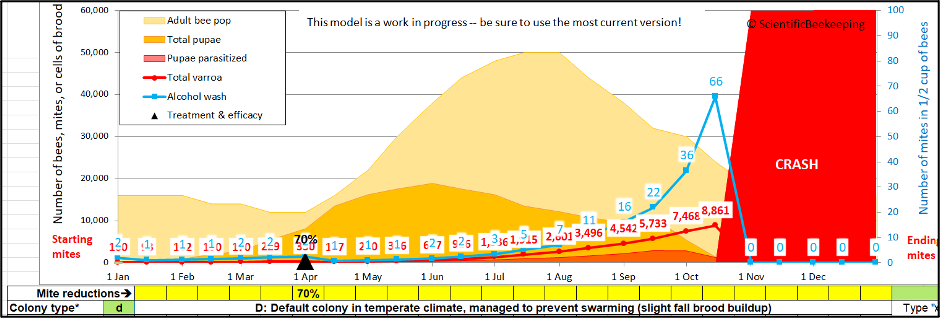
Fig.6. In this simulation of fairly-typical colony management, the efficacy of the April treatment was only so-so (due to most of the mites being in the brood). And then the beekeeper hadn’t gotten around to giving an August treatment. Going into September, the simulated colony shows a mite wash count of 16 — which would be typical of “average” management practices [[5]]. But the mite population in that hive would soon exceed 5000, meaning that an immigration of the same 165 mites as in the previous scenario would be only a drop in the bucket!
Practical application: If you’re not staying on top of varroa all season long, it may be a stretch to blame a late-season spike in mite counts upon mite immigration from elsewhere.
It appears as though mite immigration could indeed be an issue for those making the effort to maintain low mite counts. So I felt that a field study to better understand mite immigration in my operation was justified!
BACKGROUND RESEARCH
The first thing I want to do when designing a research project is to find out what is already known. For this article, I’ll snip some quotes (with minor paraphrasing and added emphasis) from relevant publications.
The second thing is then determine the specific questions that I want to try to answer — these will be my study objectives (which I will list below).
THE BIOLOGY BEHIND MITE TRANSFER BETWEEN HIVES
Varroa is a very successful parasite, rapidly reproducing in its own host honey bee colony. But for the parasite’s continued success, bloodlines of the mite must somehow “horizontally transfer” to other host colonies prior to the death of their host colony.
To the mites’ great advantage, they’re able to utilize bees exiting their host colony as an unwitting delivery service that takes them directly into other colonies within flight range. This hitchhiking not only allows varroa to infest newly-established colonies, but also to mix their genes among neighboring host colonies (which helps the mite to evolve).
HOW MUCH MITE IMMIGRATION ACTUALLY OCCURS?
It takes only a single fertile female mite to start an infestation in a colony. But beekeepers soon noticed that the degree of mite immigration was sometimes much more than that. Following Ritter’s publication, in 1988, German researchers Sakofski and Koeniger wrote a chapter called “Natural transfer of Varroa jacobsoni among honeybee colonies in autumn” [[6]]. And in 1990 [[7]] they again confirmed that colonies from which varroa had been eliminated by treatment could be reinvaded by the mite, especially during late summer and fall. And then in 1992Greatti [[8]] published data indicating stunning amounts of mite invasion taking place in his monitored colonies in Italy. These findings were followed by other research, notably that of Frey [[9]], who found that mites could drift for a considerable distance. I’ve summarize the above study results previously [[10]], but would they apply to my locations in California?
Practical application: There’s no doubt that mite migration between colonies can take place, and that it can be substantial. The questions for beekeepers then are exactly how and why it occurs, how much immigration a colony can actually receive, and is there anything that we can do to reduce it.
Study Objective #1: To determine the amount of mite immigration that actually takes place in late summer and fall where I keep my bees.
Study Objective #2: To determine whether that mite immigration is steady or episodic.
Study Objective #3: To determine whether all hives suffer equally from mite immigration, or whether some are more attractive or receptive than others.
HOW DO THE MITES GET TRANSFERRED?
Since the mites depend upon bees to carry them to other colonies, it’s important to understand this aspect. Greatti [[11]] listed the three main methods by which the mite manages to disperse from one colony to another:
- By the drifting of drones, which are known to drift freely between hives.
- By the drifting of worker bees — which will tend to “diffuse” mites from colonies with high infestation to those with low infestation.
- By the robbing of colonies weakened by heavy infestation.
HOW IMPORTANT IS THE DRIFTING OF DRONES?
A recent study by Mortensen [[12]] confirmed that drones do carry mites. It’s well known that drones tend to drift to other hives. That said, Greatti, along with quantifying the actual amount of mite immigration that could take place, tested whether placing drone excluders over the entrances of colonies would reduce immigration. He found that it did not.
Practical application: Although drones do a lot of drifting, they may not contribute much to mite drift, and since there are very few drones in our colonies in late summer, I didn’t pursue the drone factor.
HOW MUCH DRIFT IS THERE OF WORKER BEES?
There have been plenty of studies indicating that there can be considerable drift of worker bees, especially in crowded apiaries, where more than half the workers in a colony may drift at some time in their lives to other hives [[13]].
But how about the relatively sudden disappearance of the bees from a hive as it collapses from the varroa-virus complex. Do those bees just “disappear,” or do they wind up in other colonies?
Study Objective #4: To determine the proportion of workers that drift from collapsing colonies into other hives.
HOW IMPORTANT ARE THE GUARD BEES?
There’s another aspect of worker drift besides bees just getting lost — to enter a foreign hive, they first need to get past the guards. Since I have long selected for “gentle” stock, I’ve wondered whether I’ve also been inadvertently selecting for bloodlines whose guards might be more permissive to drifted bees attempting to enter their hive (Figure 7).

Fig. 7. Although we breed for gentleness, I often observe guards doing a good job at defending the entrance against intruders.
In 2017 Bordier [[14]] used video cameras to track tagged bees at the entrances of monitored hives (Figure 8).
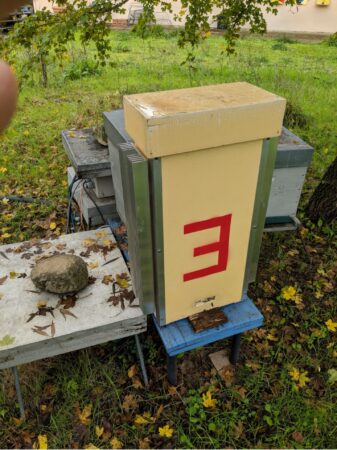
Fig. 8. I visited the INRA research facility in 2018, and snapped this picture of one of the video entrance monitoring units that they were using.
Bordier concluded that (I’ve boldfaced the highlights):
Drifting behavior is a consequence of orientation errors, and also depends on the acceptance level of the new host colony… changes in drifting intensity are likely the result of an increase in colony acceptance. In normal conditions, only 3.5 % of incoming bees are checked by guard bees, but this permissiveness changes according to season, density of bees and nectar and pollen resources— the acceptance levels of colonies increase when nectar flow is abundant but decrease during dearth periods.
Practical application: So I’m still not clear on the importance of the guards in preventing mite immigration. Of interest is the finding by Cappa [[15]] that guard bees mount a greater defense against drifting bees that have been parasitized by a mite (how much might this play into “mite resistance” of a colony?). Contrast that finding to that of Geffre [[16]], who “found that IAPV-inoculated bees were significantly more likely to be accepted by the guards at the colony entrance than were…controls.”
We obviously still have much to learn about guarding and drifting. I set up my study so that we would only recover drifted bees that made it past the guards on the landing board.
DO MITES AND VIRUSES CAUSE INCREASED DRIFTING?
It is well known to biologists that any number of parasites can change the behaviors of their hosts in order to effect better transmission [[17]]. So as proposed by DeGrandi-Hoffman [[18]] in 2017:
We hypothesize that stress from parasitism and virus infection combined with effects that viruses have on cognitive function may contribute to forager drift and mite and virus dispersal.
So is there evidence for increased drift from colonies undergoing collapse? In 1991 Sakofski [[19]] found that:
By comparing numbers of bees drifting from highly infested and noninfested small colonies it could be shown that infested colonies drifted more frequently. The invasion of mites into bee colonies was monitored during a period from spring to autumn. All colonies showed low values of mite influx which increased considerably at the end of July and remained high until the beginning of October. Individual values differed over wide ranges which are likely to be caused by robbery.
But in 2000, Neuman [[20]], although finding substantial drift of workers and drones, did not find a correlation between worker bee drift and varroa infestation
Goodwin [[21]] ran a field trial in New Zealand wrote in 2006, in which six of eight high-mite colonies with marked bees collapsed and got robbed out. Despite that:
The average percentage of bees drifting from untreated hives infested with varroa into neighboring treated hives did not exceed 3%. There were no significant differences between the percentage of drifting bees from the varroa-infested colonies and the treated colonies at any stage throughout the trial. This suggests that the reinfestation of treated colonies does not predominantly result from heavy varroa infestations causing worker bees to drift to other colonies, more than they generally do in practically varroa-free colonies.
And then in 2014 the premise that highly-infested bees were predisposed to drift was given wind by Cervo [[22]]:
At low mite abundance, mites stay within the colony where they are born and promote their reproduction by riding nurses. This preference ensures that mites are quickly transferred to another host larva within the same colony where they can reproduce. When mite abundance increases within the colony, the lack of differences in chemical cues between nurses and foragers probably does not allow mites to discriminate between bees with different tasks and causes mites to ride on both of them…. by riding both nestmate foragers and foreign [robbers], mites increase their probability of leaving the exploited colonies. An infested colony, progressively weakened by parasites and mite-transmitted diseases, finally becomes an easy target for robbing foragers from foreign colonies; these robber foreign bees might represent excellent vectors to directly transfer mites from the old exploited beehive to a new one.
This hypothesis immediately gained traction, and by 2017 the term “Mite Bombs” was being thrown about, although with little supportive evidence. And it was a foggy notion – not clearly differentiating the day-by-day “diffusive” mite drift from high-mite or collapsing colonies, versus the sudden and short-term direct transfer of mites during robbing events that may occur during the collapse of a colony.
In 2017 Bordier [[23]] found that:
Approximately 10 % of our tracked bees drifted into a foreign colony. The drifting prevalence was influenced by the colony’s location in space but not by N. ceranae parasitism.
There were no significant differences between the percentage of drifting bees from the varroa-infested colonies and the control colonies at any stage throughout the study. This suggests that the reinfestation of treated colonies does not predominantly result from heavy varroa infestations causing worker bees to drift to other colonies, more than they generally do in practically varroa-free colonies….The normal level of drift in apiaries will, however, spread varroa from untreated to treated colonies.
And in 2019 Peck and Seeley [[24]] found that:
We saw no indications that drifting of bees from the mite donor colonies into the mite receiver colonies increased as the mite levels in the mite donor colonies increased.
Practical application: As attractive as a virus-induced increase in drifting behavior hypothesis is, there are to date conflicting findings (Figure 9).
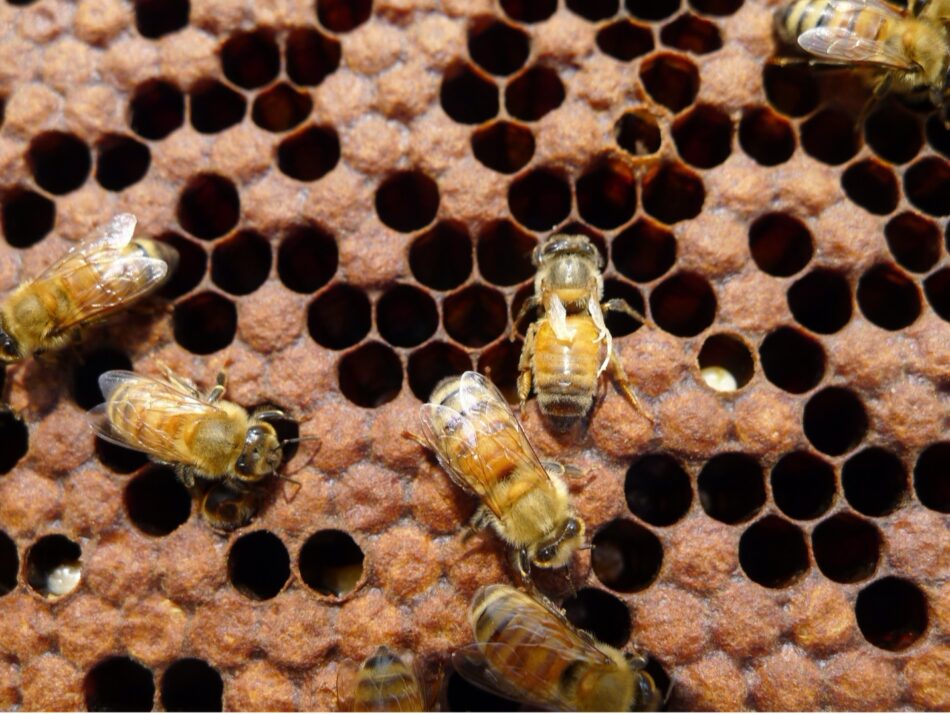
Fig. 9. It’s easy to identify an unfortunate worker that suffered from such a high infection rate of DWV that its wings could not develop. But you can safely assume that every worker in this photo is infected with DWV to some extent, as evidenced by the dying brood. But does the varroa-DWV complex cause these infected bees to drift at a higher rate?
Study Objective #5: To determine whether elevated varroa/virus levels increase bee drift in my own operation.
ROBBING VS. “ORDINARY” DRIFTING
We’ve now worked our way down to whether mite immigration (a) is primarily due to the “ordinary” drift of bees from hive to hive (which I call “mite diffusion” since the net effect will be to move mites from colonies with high mite concentration to those with lower concentration), or (b) is mainly from robbing bees carrying mites back from varroa-weakened hives. Such robbing could be surreptitious (and unnoticed by the beekeeper) or “overt” (easily observed at the entrance when a collapsing colony gets mobbed by robbers) (Figure 10).
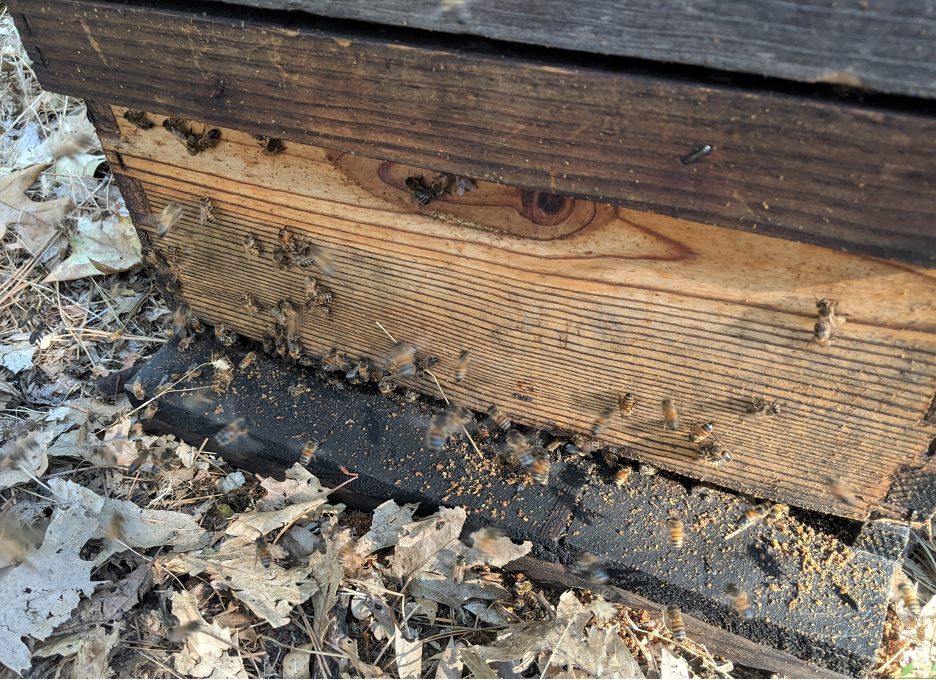
Fig. 10. The unusual activity at the entrance, coupled with the debris on the landing board indicates that this dwindled colony is getting robbed. I suspect that in our location, most mite immigration due to robbing might be from the surreptitious robbing that takes place during nectar flows, when there are few guards at the entrance.
Peck and Seeley [[25]] looked into mite drift during robbing events taking place in Ithaca, NY late in the season:
Our data also reveal that the colloquial terminology for [heavily infested] colonies—“mite bombs”—does not accurately describe the mechanisms of intercolony mite transmission that we observed in this study. We saw no sudden “explosion” of mite-carrying bees from sick colonies to each of the healthy colonies via worker drifting. Indeed, we found that few mites passed from the heavily infested (MDC) colonies to the weakly infested (MRC) colonies through either worker drift (mostly to nearby colonies) or drone drift. It was only when the MDCs were weakened so much that they became irresistible robbing targets that mites passed in large numbers from the MDCs to the MRCs.
Practical application: The mites in a colony that is being robbed as it is undergoing collapse (while there are still plenty of live mites in the hive) can certainly hop onto the robbers (which the mites could easily recognize due to their different odor) and get carried back to the robbing colony. But such immediate robbing doesn’t take place in all landscapes — see my ancillary article in this issue.
And finally, Kulhanek published a study last year [[26]].
More bees from low mite colonies (n= 37) were detected in receiver apiaries than bees from high mite colonies (n= 10). Receiver colony Varroa population growth was associated with visitation by non-natal bees, but not high mite bees alone. Finally, colonies lacking robbing screens experienced faster Varroa population growth than screened neighbors. Results indicate visiting non-natal bees may vector mites to receiver colonies. These results do not support the current two leading theories regarding mite immigration – the “mite bomb” theory (bees from high mite colonies emigrating to collapsing colonies), or the “robbing” theory (natal robbing bees return home with mites from collapsing colonies).
Practical application: Although mite drift during rob outs can indeed take place, it may be that mite diffusion due to ordinary bee drift may be more important. Since robbing screens don’t prevent colonies from robbing, Kulhanek’s findings suggest that they may reduce bee (and therefore, mite) drift (teaser: I will soon be publishing my own testing of robbing screens).
Study Objective #6: To determine whether mite immigration correlates with robbing.
There’s still one more item of interest…
HOW FAR CAN MITES HITCHIKE A RIDE?
A study by Frey in 2011 [[27]] had a surprising finding — that mite drift is not necessarily a function of distance, and that there can be considerable drift even to colonies a mile distant.
During the experimental period, between 85 and 444 mites per colony were introduced into the receiver colonies. There were no significant differences in the invasion rates in relation to the distance between donor and receiver colonies. In total, 2,029 mites were found in the 10 receiver colonies, but these only correspond to 2.5% of the total mite population in the donor colonies at the start of the experiment. This means that the major part of the initial V. destructor population died together with the collapsed host colonies…From a practical perspective we could show that highly infested honey bee colonies present a substantial risk to already treated colonies up to distances of 1.5 km [roughly a mile] away.
Kulhanek [[28]] observed substantial drift between hives in the same yard, but her cameras observed only 47 marked bees (out of approximately 32,000 painted) drifting to receiver hives a half mile or further distant.
Practical application: Frey’s finding of the lack of correlation between mite drift and distance was something that I wanted to test in my home yard.
Study Objective #7: To determine the distance that bees can drift from collapsing colonies.
Study Objective #8: To determine how the rate of drift to nearby hives compares to that to hives more distant.
NEXT MONTH
In the next installment I’ll show we set up our field study in order to find out the answers to the listed objectives. Stay tuned!
ACKNOWLEGEMENTS
Thanks to Peter Borst and Dianne Behnke for their assistance with finding and scanning copies of old research papers.
CITATIONS AND NOTES
[1] The Varroa Problem: Bee Drift and Mite Dispersal, Parts 16 a & b:
https://scientificbeekeeping.com/the-varroa-problem-part-16a/
https://scientificbeekeeping.com/the-varroa-problem-part-16b/
[2] Ritter, W., & E Leclercq (1987). Entwicklung der Bienen-und Varroa-population in Gebieten mit unterschiedlichen Möglichkeiten der Reinvasion. Tierärzt Umschau 42: 548-51.
[3] https://scientificbeekeeping.com/scibeeimages/Randys-Varroa-Model-V2022-1-1.xlsx
[4] This figure is calculated from the calculated number of non-returning adult bees per day (due to aging, getting lost, or other causes), assuming that they are carrying mites at only 2/3 the infestation rate of the worker bees on average).
[5] Kulhanek K, et al (2021) Survey-derived best management practices for backyard beekeepers improve colony health and reduce mortality. PLoS ONE 16(1): e0245490.
[6] Sakofski, F & N Koeniger (1988) Natural transfer of Varroa jacobsoni among honeybee colonies in autumn. In European Research on Varroatosis Control.
[7] Sakofski, F, N Koeniger, S Fuchs (1990). Seasonality of honey bee colony invasion by Varroa jacobsoniOud. Apidologie, 21(6), 547-550.
[8] Greatti, M, N Milani, F Nazzi (1992). Reinfestation of an acaricide-treated apiary by Varroa jacobsoniOud. Experimental & applied acarology, 16(4), 279-286.
[9] Frey, E, H Schnell, P Rosenkranz (2011). Invasion of Varroa destructor mites into mite-free honey bee colonies under the controlled conditions of a military training area. Journal of Apicultural Research, 50(2), 138-144.
[10] https://scientificbeekeeping.com/the-varroa-problem-part-16b/
[11] Greatti, M (1992) Op. cit.
[12] Mortensen, A, C Jack, J Ellis (2018). The discovery of Varroa destructor on drone honey bees, Apis mellifera, at drone congregation areas. Parasitology Research 117(10): 3337-3339.
[13] Pfeiffer, K & K Crailsheim (1998). Drifting of honeybees. Insectes Sociaux 45(2): 151-167.
[14] Bordier, C, et al (2017) Should I stay or should I go: honeybee drifting behaviour as a function of parasitism. Apidologie 48(3): 286–297.
[15] Cappa, F, et al (2016) Bee guards detect foreign foragers with cuticular chemical profiles altered by phoretic varroa mites. Journal of Apicultural Research 55(3): 268-277.
[16] Geffre, A, et al (2020). Honey bee virus causes context-dependent changes in host social behavior. Proceedings of the National Academy of Sciences, 117(19): 10406-10413.
[17] Poulin, R (2010) Parasite manipulation of host behavior. Advances in the Study of Behavior 41: 151-186.
[18] DeGrandi-Hoffman, G, F Ahumada, H Graham (2017). Are dispersal mechanisms changing the host–parasite relationship and increasing the virulence of Varroa destructor (Mesostigmata: Varroidae) in managed honey bee (Hymenoptera: Apidae) colonies? Environmental Entomology 46(4): 737-746.
[19] Sakofski, F (1991) Quantitative investigations on transfer of Varroa jacobsoni Oud. In Recent research on bee pathology. International symposium of the International Federation of Beekeepers Associations, Gent (Belgium), 5-7 Sep 1990.
[20] Neumann, P, et al (2000) Colony evaluation is not affected by drifting and worker honeybees (Apis mellifera L.) at a performance testing apiary. Apidologie 31: 67–79.
[21] R M Goodwin, et al (2006) Drift of Varroa destructor-infested worker honey bees to neighbouring colonies. Journal of Apicultural Research 45(3): 155-156.
[22] Cervo, R, et al (2014) High Varroa mite abundance influences chemical profiles of worker bees and mite-host preferences. J. Exp. Biol. 217: 2998– 3001.
[23] Bordier, C, (2017) Op. cit.
[24] Peck DT & TD Seeley (2019) Mite bombs or robber lures? The roles of drifting and robbing in Varroa destructortransmission from collapsing honey bee colonies to their neighbors. PLoS ONE 14(6): e0218392.
[25] Peck DT (2019) op. cit.
[26] Kulhanek, K, et al (2021) Accelerated Varroa destructor population growth in honey bee (Apis mellifera) colonies is associated with visitation from non-natal bees. Scientific reports, 11(1): 1-15.
[27] Frey, E (2011) Op. cit.
[28] Kulhanek, K (2021) op cit.
Contents
Questions to Answer 1
Materials and Methods 2
Placement of the hives 2
Preparation of the Mite Donor Colonies. 3
Tagging the bees 3
The Control Group. 7
Preparation of the Mite Receiver Hives 7
Eliminating the mites. 7
Magnetic tag recovery. 9
The stickyboards. 10
The hive scales. 10
Layout of the Donor hives 11
Acknowledgements And an Apology. 12
Citations and Notes 12
A Study on Bee Drift and Mite Immigration
Part 2
Beekeeper-funded applied research – by a beekeeper, for the benefit of beekeepers.
Randy Oliver
ScientificBeekeeping.com
First published in ABJ March 2023
Previous research indicates that varroa mites utilize drifting and robbing bees to get carried to other hives in the neighborhood. But there were still a number of outstanding questions about the details of mite immigration. Wanting more information, in 2018 I undertook an ambitious study to answer a few.
Back in 2018, beekeepers were talking about “mite bombs,” and blaming their problems on the immigration of mites from highly-infested neighboring colonies outside of their control. Last month I reviewed published studies regarding this phenomenon. But we must keep in mind that institutional researchers are often under pressure to “publish or perish.” In this competitive environment, I unfortunately see a lot of conclusions being drawn from datasets involving a relatively small number of marked bees and hives.
Wanting to gain a better understanding of bee drift and mite immigration in my own operation, I decided to run a larger-scale study, involving more colonies, more marked bees, and greater distances, in order to help answer the following eight questions:
Questions to Answer
- How much mite immigration actually takes place in late summer and fall?
- Is mite immigration steady or episodic?
- Does mite immigration correlate with robbing?
- Do all hives suffer equally from mite immigration, or are some more attractive to drifting bees?
- What proportion of workers from collapsing colonies drift to other hives?
- Do elevated varroa/virus levels increase bee drift from a hive?
- How far do bees from collapsing colonies drift?
- How does the drift of bees to nearby hives compare to that of those more distant?
Could I possibly get answers to all the above questions from a single field study? Since the devil is always in the details, in this article I’ll go over how we set up the study.
Materials and Methods
At my home in the dry Sierra Foothills [[1]], we set up ten “Donor hives” (containing tagged bees) to intentionally collapse from varroa (unfortunately one collapsed prematurely), and then twenty-four “Receiver hives” at various distances to determine the number of tagged bees drifting into them from the collapsing Donor colonies (Figures 1 & 2).
Placement of the Hives

Fig. 1 The layout of the home yard, showing placement of the collapsing Donor hives, as well as the Receiver Hives from which data for bee drift and mite immigration was taken. We are surrounded by sloping mixed oak/pine forest, with irrigated pasture to the east. My garden and orchard are in the middle of the photo. The brown dry ground is how our non-irrigated land looks during the summer.

Fig. 2 Zooming out, with my home yard indicated by the golden star. We monitored six surrounding apiaries of ours (red crosses) for drifted bees. Each apiary held around 30 colonies, although only three vigorous colonies in each were prepared as “receivers.”
Study design: The above setup allowed us to answer the questions “How far do bees from collapsing colonies drift?” and “How does the drift of bees to nearby hives compare to that of those more distant?”
Preparation of the Mite Donor Colonies
Following a standard practice of using colonies of “black” or “yellow” bees to identify those that drift, in June we prepared two different sets of hives with bees of different colors. We requeened the Donor Hives (intended to collapse) with “yellow” cordovans, and the mite-free Receiver Hives with dark Carniolans (graciously provided by queen producers Frank Pendell and Valeri Severson of Strachan Apiaries respectively).
Over the course of July we fed the colonies to grow them, boosting them if appropriate with combs of high- or low-mite brood. At the end of July we took mite wash counts again — some of the Donors already had counts of 50-100 mites per half cup of bees! So we replaced some brood combs with those from low-mite colonies, and hit them with half a MAQS formic pad, in order to keep them from collapsing before we had the Receiver hives ready.
Tagging the Bees
It became apparent that due to the swapping of combs, that the black/yellow scoring method of bees for parent colony identification wasn’t going to work, so after reviewing various alternative methods for marking bees, I decided to instead modify a clever bee-tagging method developed years ago by the inventive Dr. Norm Gary [[2]], whom I’ve known for years, and who was happy to help me with details. My helper Brooke Molina and I spent the latter half of July and into August perfecting the technique, figuring out the best anesthetization method, holders for the bees, type of glue, etc. (Figure 3).
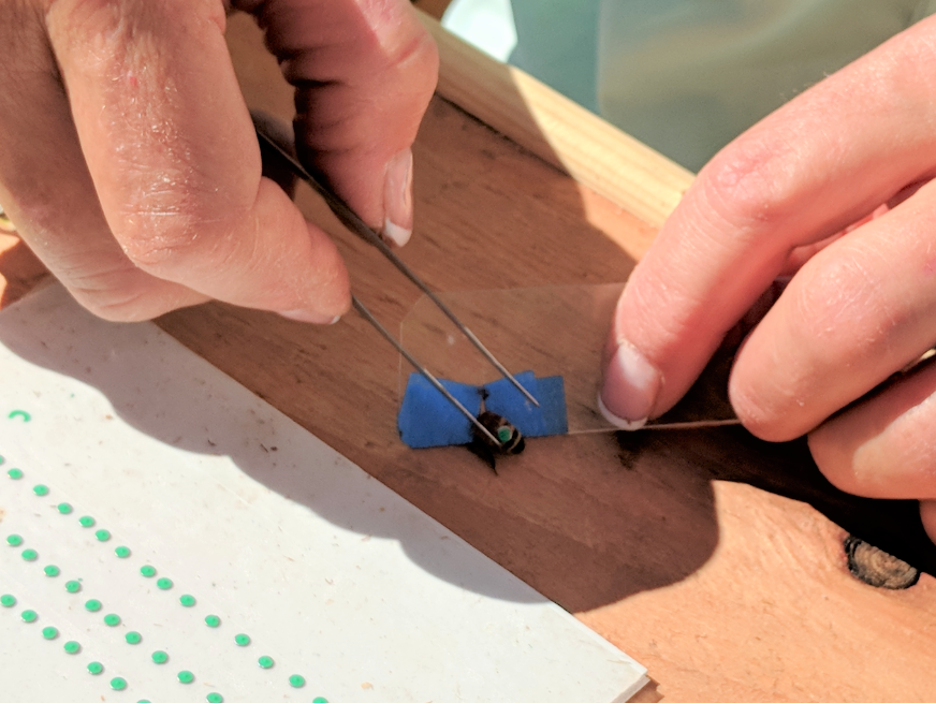
Fig. 3 We hand-punched over 6000 thin steel discs and Brooke hand-painted them with different colors for each Donor colony, placing them on cards covered with double stick tape. The tagging crew then glued them onto an average of 540 bees per colony during each Donor’s collapse. Here’s Alice Dunbar demonstrating how we used a piece of plastic with a V-notch (wrapped over with masking tape) to slide over a bee’s petiole, and harmlessly hold it still with its wings out of the way. We put a drop of Elmer’s quick-drying glue on the abdomen, then used forceps to drop and tap a disc into the glue.
Alice’s husband Brion filmed her tagging bees; you can watch her do it at https://youtu.be/2FEeL-O08qA.
Experimental note: After experimentally confirming Dr. Gary’s suggestions for disc thickness and diameter, we punched 0.09” diameter discs from 0.010” sheet steel. The painted discs weighed less than 10 mg — only a fraction of the weight of a load of nectar. We also followed his suggestion [[3]] that “Label attachment on the abdomen seems preferable to thoracic attachment for several reasons. There is less risk of accidentally fouling the wings with adhesive. Furthermore, labels tend to break free from the abdomen more easily because there are fewer cuticular setae. The abdomen is adapted for carrying nectar or water loads that constitute the heaviest weights transported by bees. Attachment of inflexible metal label to the thorax possibly may affect flight behavior, either by creating a balancing problem or by adversely affecting the scutum’s flexibility.” We were also careful to avoid magnetizing the discs, so as not to affect the bees’ magnetoreception during flight [[4]].
For tagging the bees, I custom built a chair with an adjustable desk to each of my helpers’ preferences. Some of the taggers just loved sitting outside and enjoying the scenery while they glued on tag after tag (Figure 4).
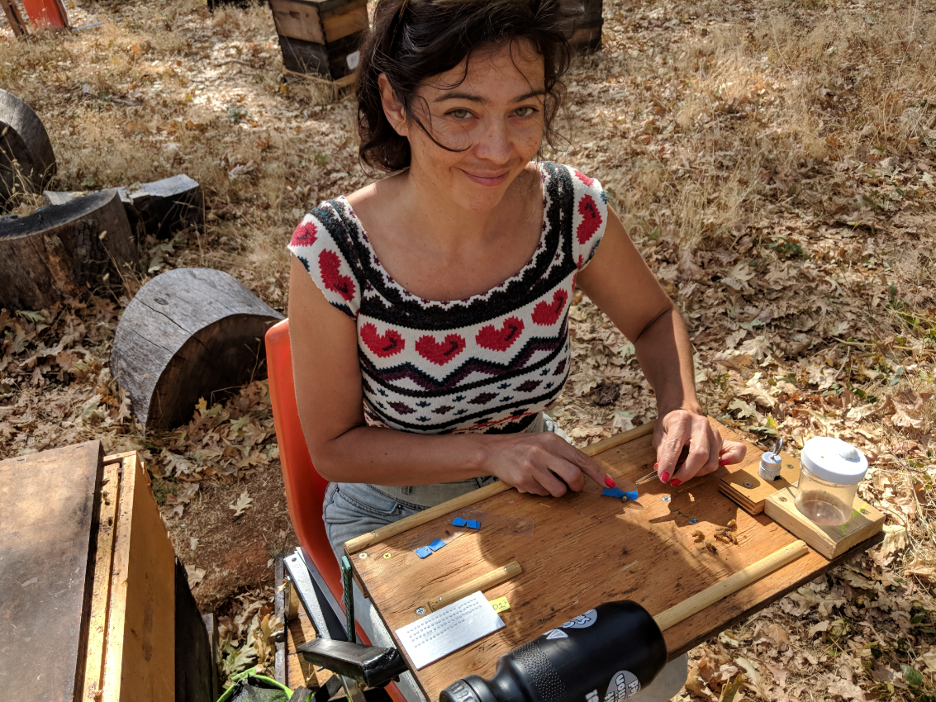
Fig. 4 Here’s Brooke sitting next to a hive, marking bees. We behaviorally-sorted young workers to tag by dumping shook bees into the black bottles — the older bees immediately flew out toward light, whereas young workers remained inside in the darkness. The tagger shook out as many bees as they could process in a few minutes into the clear plastic bottle, and then gave it a shot of CO2 (using a bicycle tire filler with a hollow needle) to briefly anesthetize them.
It takes a number of hands to tag 6000 bees, so in addition to Brooke and the Dunbars, we were helped by Anna Mudd. Some of us were quicker at it than others, so I paid my helpers by piece rate (Figure 5).

Fig. 5 Anna had been grafting queens for us so her fine-motor skills were well developed. I snapped this photo when she realized that she could earn more per hour tagging bees than she’d ever made in any previous job.
As we glued tags on the bees, we sat next to the hive, with the hive cover slid partially open, so that we could immediately return each tagged bee back to its colony (Figure 6).

Fig. 6 The taggers immediately placed the tagged bees onto the top bars of the hive from which they had been taken. The bees quickly recovered from the anesthetization, walked down onto the combs, and there acted normally.
The Control Group
In order to answer the question “Do elevated varroa/virus levels increase bee drift from a hive?” I needed to run tagged mite-free Control colonies to compare their worker drift to that from collapsing colonies. I placed two treated mite-free Control hives within the high-mite Donor group of hives. Spoiler: As you’ll eventually see, I should have run as many Controls as Donors. (By good fortune I got lucky. Sometimes that happens).
Preparation of the Mite Receiver Hives
Eliminating the Mites
In order to quantify the amount of mite immigration into the Receiver hives, we copied the methodology of Sakofski [[5]], Greatti [[6]], and Frey [[7]], in which they used high-efficacy synthetic miticides to eliminate the mite population in receiver colonies. This allows the creation of virtually mite-free colonies (exhibiting daily mite drops and mite washes of zero). Continual treatment with slow-release miticide strips then causes any incoming mites (carried by bees) to drop within a day onto a stickyboard, providing a count for mite immigration.
Since we hadn’t used any synthetic miticides for over 15 years (and rotate a quarter of our brood combs each year), our mites exhibit no resistance to experimentally-applied amitraz or fluvalinate, so we applied those two active ingredients (Figure 7).
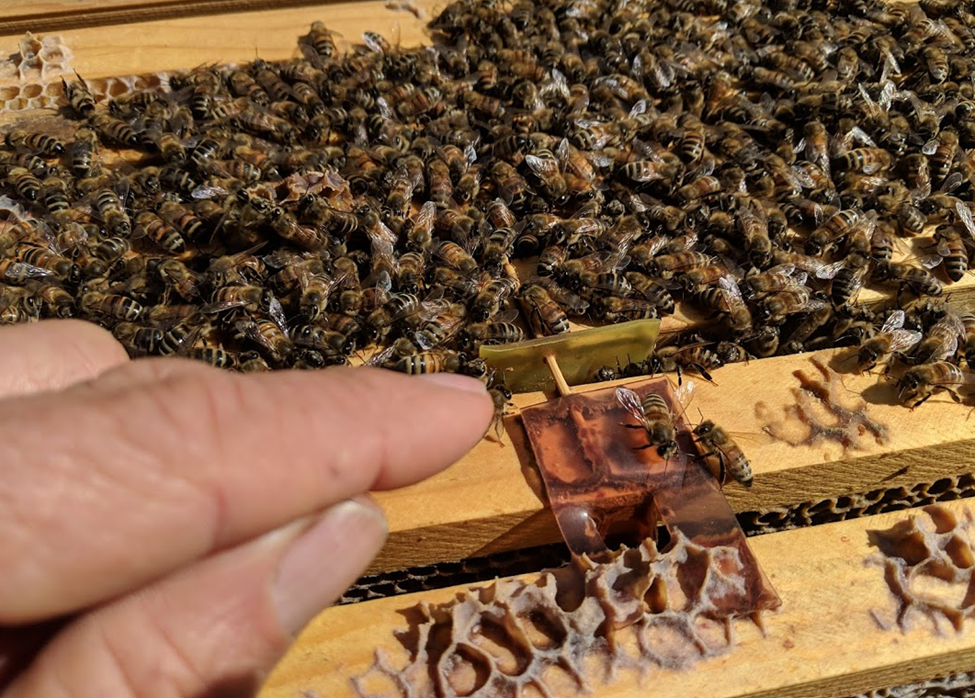
Fig. 7 We started the colonies in April 2018, treating them with oxalic acid, then in June with formic acid, followed by a combination of Apistan plus Apivar strips.
The prepared Receiver hives in the home yard thus were mite-free, continually treated, with stickyboards, scales, and magnetic entrance traps (Figures 8 & 9). We didn’t place stickyboards or scales on the outyard Receiver hives.

Fig. 8 A Receiver hive ready to go! I designed the entrance traps so when a drifted tagged bee entered the hive, its steel tag would snap onto the magnet very firmly. I set the entrance height so that the stuck bee could still grab the bottom board with its feet and pull itself free of the color-coded tag — leaving a clear record of the bee’s entry that we could then later record without having to be there to observe the actual event.
Magnetic Tag Recovery
After consultation with Norm Gary, I designed and fabricated magnetic tag retrieval entrance traps, improved by using super-strong neodymium magnets not available when he invented the method [[8]] — they worked great! A magnetic trap placed at the entrance of each Receiver hive removes and holds the tag of any drifted bee that attempts to enter it, allowing us to go out every couple of days and quickly remove and record any tags from drifted bees at each colony entrance. The beauty, for my purposes, of using Dr. Gary’s ferrous tagging technique is that it not only records the entrance of a drifted bee, but also its home colony.
Practical application: Although state-of-the art methods of tracking bees with RFID tags or video recording are wonderful, Norm’s tried-and-true “old school” technique works extremely well and is essentially foolproof, since it collects hard evidence in the form of painted discs that can be recovered at the investigator’s convenience, rather than by sitting in front of a hive (or a video) looking for returning bees, or opening the hive and searching for marked bees on the combs. I’m surprised that more researchers don’t use it!
The Stickyboards
We installed rear-access stickyboards under screened bottom boards in all the Receiver hives in the home yard (Figure 9). We recorded the number of dropped mites roughly every third day.
Study design: The stickyboard counts of the Receiver hives could help us to answer the following three questions:
How much mite immigration takes place in late summer and fall?
Is mite immigration steady or episodic?
Do all hives suffer equally from mite immigration, or are some more attractive to drifting bees?

Fig. 9 I make sturdy stickyboards out of FRP (fiber-reinforced plastic) wall paneling, which can be used and reused for years. After counting and recording the number of mites, we scrape off the adhesive, mites, and hive trash with two strokes of a sheetrock knife. We then re-roll on a mineral oil/ petroleum jelly mixture with a small-diameter paint roller, and reinsert the stickyboard.
The Hive Scales
In order to track whether a Donor colony was being robbed, or a Receiver colony was robbing other hives, I put Broodminder electronic scales under both the Donor and Receiver hives in the home yard. Thanks to Rich Morris of Broodminder for cutting me a deal on the scales, and for his help with setup and data processing. I found the Broodminder scales to work very well for my purposes. I confirmed that placing a single scale under the front end of the bottom board accurately reflected total hive weight change.
Study design: In order to answer the question of whether mite immigration correlates with robbing (in our foothill environment), I intentionally performed this study during our late-season nectar dearth (when no weight gain would be taking place). A robbing colony would exhibit weight gain, whereas a colony being robbed would show a weight loss. The stickyboards would allow us to determine how many mites were getting rides into the hive on any day. We could then look for correlations.
Layout of the Donor hives
Figure 10 shows the layout of the donor and close Receiver hives.
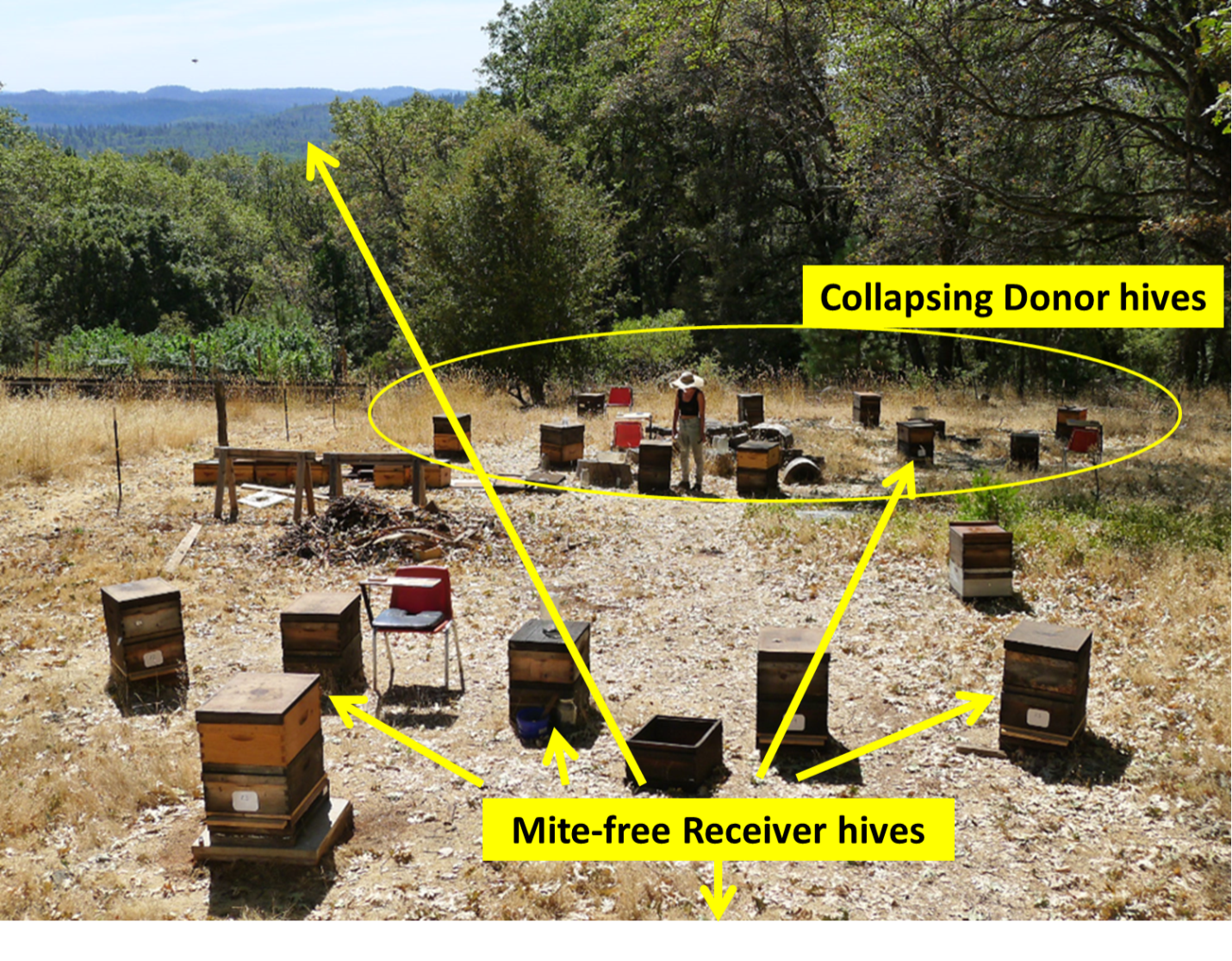
Fig. 10 The view looking south from my back porch. The long arrow points to our nearest apiary a half mile away. We’re in Northern California, so the requisite solar panels and cannabis garden are in the background. The down arrow points towards my house, with more Receiver hives some 500 feet away.
OK, I’ve shown how we set up a study to attempt to obtain answers to all eight our original questions. I’ll continue with our findings next month.
Acknowledgements And an Apology
I greatly appreciate the help from my crew — Brooke Molina, Anna Mudd, and Brion and Alice Dunbar, without whose help I could never have pulled this study off. Also a shout out to Dr. Norman Gary, whose impressive body of research has been instrumental to mine.
I again thank Valerie Severson and Frank Pendell for the queens, and Rich Morris for help with the Broodminder scales.
Last but not least I thank those beekeepers whose donations help me cover the costs of my applied research projects. That said, I apologize for waiting so long to publish my results, but at the time of this project I was chastised by a university lab leader who was upset that I kept getting the jump on their own research projects. So out of courtesy I’ve delayed presenting my findings until they published theirs.
Citations and Notes
[1] GPS coordinates 39.17702, -120.99153, 2880 ft elevation, yellow pine/black oak woodlands.
[2] Gary, N (1971) Magnetic retrieval of ferrous labels in a capture-recapture system for honey bees and other insects. J. Econ. Ent. 64: 961-965.
[3] Ibid.
[4] Liang, CH, et al (2016) Magnetic sensing through the abdomen of the honey bee. Sci. Rep. 6: 23657.
[5] Sakofski, F., Koeniger, N., & Fuchs, S. (1990). Seasonality of honey bee colony invasion by Varroa jacobsoni Oud. Apidologie, 21(6), 547-550.
[6] Greatti, M, N Milani & F Nazzi (1992) Reinfestation of an acaricide-treated apiary by Varroa jacobsoni Oud. Experimental & Applied Acarology 16(4): 279-286.
[7] Frey, E., H Schnell & P Rosenkranz (2011) Invasion of Varroa destructor mites into mite-free honey bee colonies under the controlled conditions of a military training area. Journal of Apicultural Research 50(2): 138-144.
[8] Magnet size 3 x 6 x 60 mm, set in line in a groove.
Supplementary material for A Study on Bee and Mite Drift, Part 5
Due to unfamiliarity with data preservation for the scale hives, we lost all our raw data when we removed the batteries from the scales at the end of the trial. Luckily, I had been following the weight data during the course of the trial, and had taken a few screenshots. I fully expected to see evidence of robbing correlating with mite immigration, but did not.
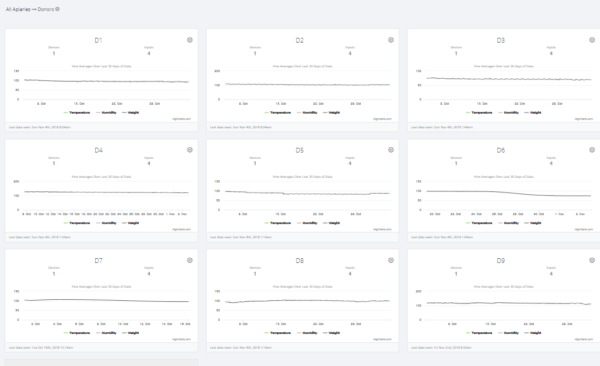
A screenshot of scale data for the Donors.The only sudden weight losses that we observed were for Donors D6 and D8 (not shown in this chart) ― the rest of the Donors showed no indication of getting robbed during their collapse. But the bulk of those weight reductions may well have simply represented the body mass of the workers that abandoned the hive (the bees covering 8 combs weigh roughly 4 pounds).


A screenshot of typical scale data for the Receivers, with weight gains by R4 and R7.
Contents
The Fabrics to Test
Preparation
A Field Trial
Results
Discussion
TESTING COTTON MATRICES FOR OAE
Randy Oliver
ScientificBeekeeping.com
First published in ABJ January 2023
I continue to look for improved methods for application of extended-release oxalic acid (OAE), and thought that perhaps there might be an inexpensive, fully biodegradable all-cotton fabric that might do the trick.
Chipboard (cardboard) strips work well for hung-over-the-frame application of OAE, but it takes a lot of time to insert enough of them to attain good efficacy, plus they need to be repeated. I’ve already found that Swedish cellulose sponges work very well for top bar application of OAE, but they’re kinda pricey. Cotton looked promising in some of my preliminary testing last season, so I decided to follow up on absorbent cotton fabrics.
THE FABRICS TO TEST
Having little familiarity with what sorts of cotton fabrics are available, I checked online, at fabric stores, and in thrift shops. Many cotton fabrics also contain polyester, but since such plastics are not easily biodegradable, I stuck with 100% cotton only.
I finally came up with three such fabrics to test (Figure 1) — terrycloth, sweatshirt fleece, and (at the suggestion of beekeeper who had used it successfully) cotton batting. I ordered several different battings for preliminary testing, and found one (Pellon Wrap-N-Zap) that had enough fiber strength to hold together during handling, was 100% cotton, and very inexpensive in bulk. I had high hopes that I’d found the ideal matrix!

Fig. 1 End views of the three tested cotton matrices, compared to a Swedish sponge. I found out that it is very difficult to find a pure cotton sweatshirt fabric, so the one that I tested was thinner than I’d hoped for.
PREPARATION
I cut 10 pads of each fabric, all to the same dimensions as a half Swedish sponge, weighed them, and then saturated them at the same time from a single batch of heated oxalic-glycerin solution (Figure 2).
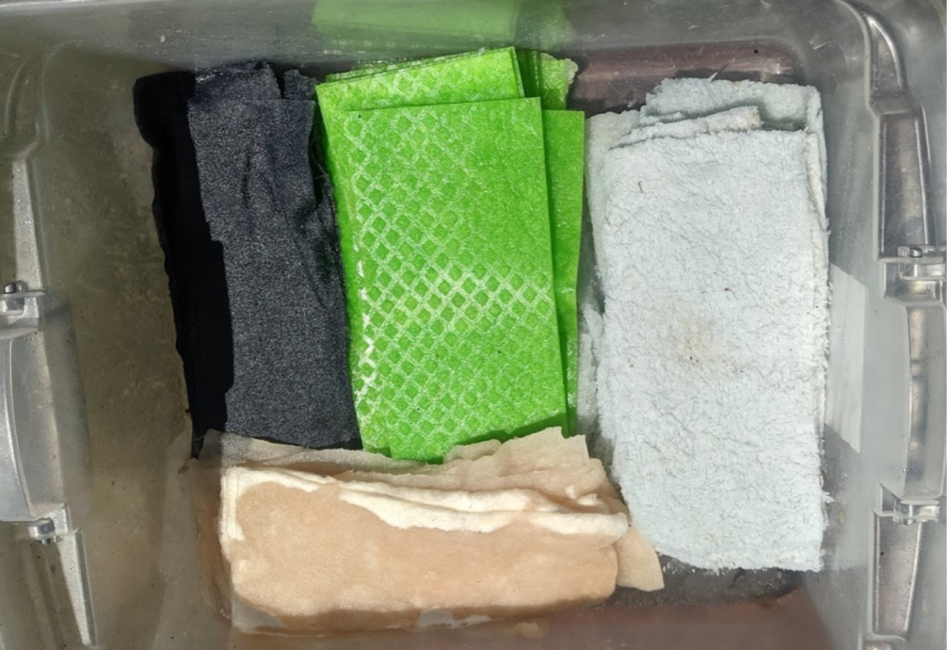
Fig. 2 I cut all the pads to match the dimensions of Swedish sponges cut in half. After sitting overnight, the batting pads (at bottom) showed some irregularity of saturation on their surfaces, but their handling characteristics were OK once the oxalic crystallized.
I then reweighed the pads to determine the average absorption of OA solution by each matrix, so that I could compare it to that of the sponges (Table 1).
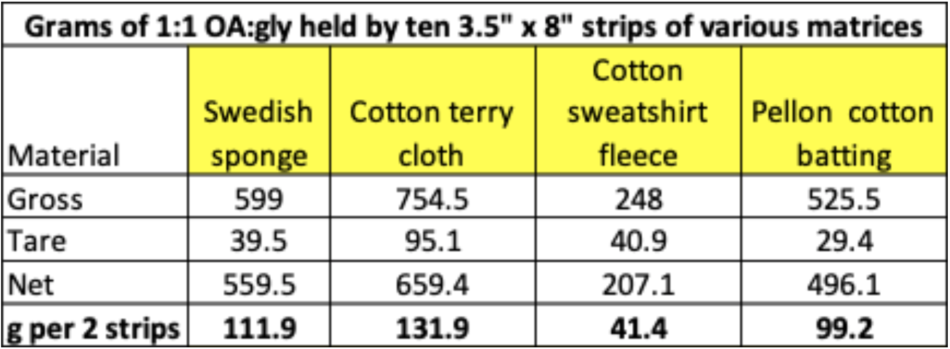
Table 1 Absorption of oxalic-glycerin solution by the matrices. Compared to the sponges, terrycloth held more solution, the batting about the same amount, but the sweatshirt fabric far less.
I had really hoped that the batting would be the dream matrix that I’ve been looking for: cheap, biodegradable, no additives. Unfortunately, I found that this batting was initially resistant to the absorption of water or glycerin (Figure 3).
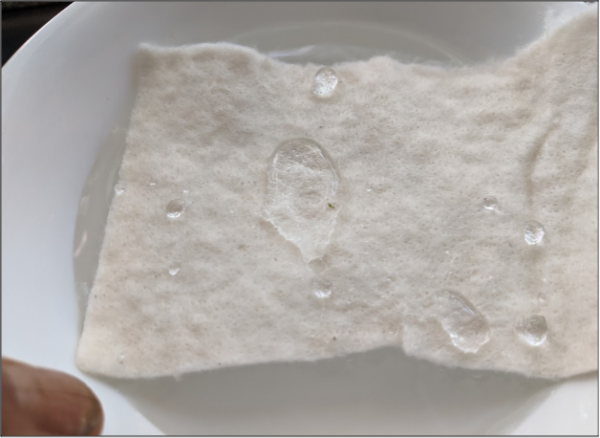
Fig. 3 The cotton batting looked ideal, until I dribbled it with water. Unlike the Swedish sponges or terrycloth, which are treated for rapid absorption of water, the batting was not, and took substantially longer to wick up the heated oxalic-glycerin solution (strike one against it).
A FIELD TRIAL
We had a couple of yards of colonies in late May with moderate to high mite counts, so we took starting mite wash counts, and randomly assigned the different matrices, blocked by starting mite count (Figure 4).
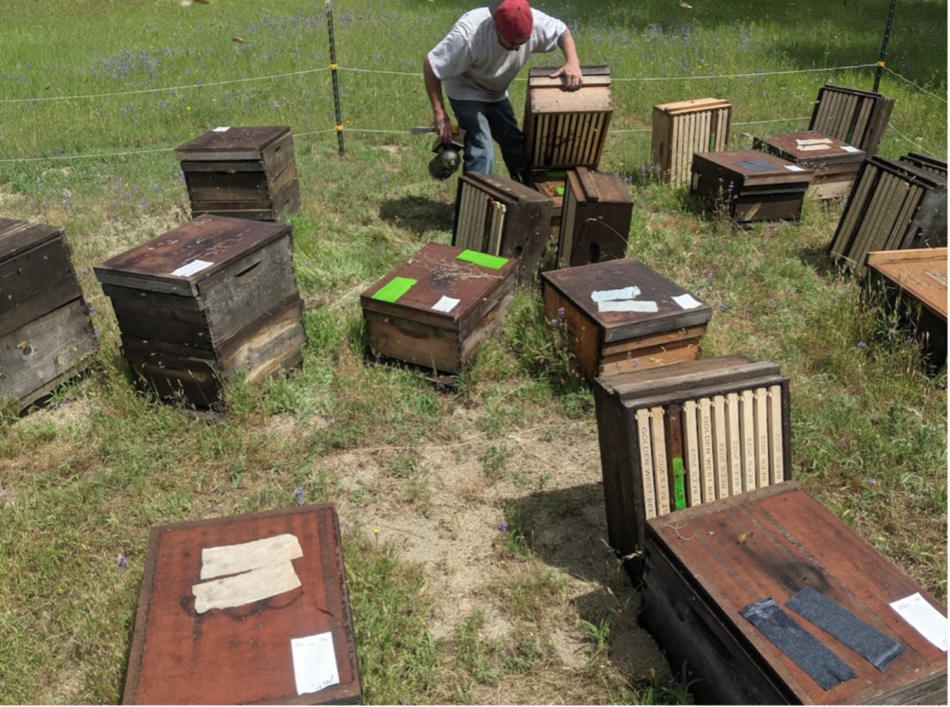
Fig. 4 On 20 May we applied the pads to double-deep medium-strength hives, replicated in two different yards (which turned out to be a good decision). Here are the pads laid out on top of the hives in Yard D before application. We left the colonies alone, and took final mite counts on 25 July (66 days later).
RESULTS
As is often the case in field trials, we got differing results in the two yards (which is why it is always best to replicate trials). To our surprise, some of the hives with the highest starting counts (28, 31, 42, 49, and 63 mites per half cup of bees) in Yard D collapsed, equally in all treatment groups.
Practical note: In our previous trials in which we applied OAE treatments later in the season, they’ve always managed to turn even very high mite infestation rates around and save the colonies. On the other hand, the few times that I’ve applied OAE treatments to high-mite colonies in the springtime, some of them have collapsed. I don’t know whether that means anything, so as they say, “Further testing is necessary.”
None of the colonies collapsed in Yard L, likely because they had also started with lower mite counts. But there were other differences between the two yards:
- The colonies in Yard L had enjoyed a good nectar and pollen flow, and gained weight. At the end of the trial, all the matrices looked moist on their upper surfaces, and after a light touch with my fingertip, I was able to taste strong acidity. When I placed samples of the spent matrices into zipper lock bags, there were droplets of oxalic solution on the insides of the bags.
- In Yard D, on the other hand, the landscape looked drier. There had been very little honey flow, the colonies were weak, and the surfaces of all the matrices — other than the sponges — were not looking moist. When I touched them with a fingertip, I did not detect as much acidity. And when I placed samples of spent matrices into zipper lock bags, no droplets showed.
Practical application: The differences in the appearances of the upper surfaces of the matrices in the two yards really stood out. The main difference between the yards that stood out was that Yard D was drier and enjoyed less nectar flow, but I have no idea why that would cause there to be less OA-glycerin solution on the upper surfaces of the matrices, since our tests in previous years have been run under very dry conditions. Why the cotton matrices would perform differently than the Swedish sponges I don’t know.
As far as mite reductions after the treatments, the yard-to-yard differences were also substantial, and strongly correlated with how “dry” the matrices appeared. Mite control was good for all matrices in Yard L (Figure 5).

Fig. 5 Mite control was good for all matrices in Yard L. I didn’t run untreated controls, so can only show starting (blue) and ending (red) mite counts for each colony. There were similar mite reductions for all matrices: median reductions 92% for the cotton felt, and 84% for all three of the other treatments.
It was a different story in Yard D (Figure 6).

Fig. 6 The median reductions for the surviving colonies were not nearly as good in this yard, as indicated by the greater amount of red showing. Median mite counts actually went up for the cotton batting, and the median reductions were 21% for the felt and 38% for the terrycloth. The only matrix that worked well in this yard was the sponges, which exhibited a median reduction of 82%.
DISCUSSION
These results were a great surprise to us. I dare not attempt to explain why some of the treatments failed in Yard D, since later in the season, when conditions were dry, OAE across the rest of our operation (applied on shop towels followed by Maximizer pads) provided excellent mite control.
The sponges were clearly the standout for success (we didn’t include Maximizers, which I suspect would have performed similarly). But the abject failure of the cotton matrices in general (and especially the batting) in Yard D have soured me on cotton fabrics.
I’ll continue to test other inexpensive matrices. If you have had success with a biodegradable matrix that does not contain questionable additives (check the product’s Material Safety Data Sheet), please let me know.
I’m hearing positive feedback on OAE from all over, and will soon be writing more about my research this past season.
Contents
Robbing is Governed by Olfaction
Back to what happens upon colony collapse
A Survey on Whether Robbing Takes Place at Collapse
My Conclusions From the Survey Responses
Relationship to Bee and Mite Drift
A humorous anecdote regarding training bees to a scent
Acknowledgements
Citations and notes
A SURVEY ON ROBBING AT COLLAPSE
Randy Oliver
ScientificBeekeeping.com
First published in ABJ February 2023
Peck and Seeley studied mite drift in an apiary in Ithaca, NY, and determined that the robbing of collapsing hives played a major role in producing surges in the mite loads of the robber colonies. But that didn’t seem to be the case where I live.
Honey bee researchers must be careful about extrapolating their field observations to other environments. The above researchers, aware that I had recently wrapped up a field study of my own, honored me by asking me to review a preprint of their paper (based upon David Peck’s doctoral dissertation). I shared my observations and data with them, and we discussed our findings at length. It became clear that although robbing during the collapse of colonies indeed occurs in the location where they made their observations, that it doesn’t necessarily happen that way in other areas.
Our discussion got me thinking about why this difference occurs. What came to the fore is just how importantly scent is involved in robbing behavior.
ROBBING IS GOVERNED BY OLFACTION
Some years ago, a friend and I built a six-sided screened enclosure for our county fair, in which we could perform a hive inspection demo each day — directly in front of viewers — with the bees coming and going freely through the open top of the enclosure, well above head level (Figure 1). This demo method has been a resounding success for presenting beekeeping to the public, since the audience can watch the presenter work bees without protective gear, while they stand inches away on the other side of the black screen.
Compared to looking through the glass of our observation hives in a nearby booth, watching a beekeeper open a hive directly in front of their faces is an entirely different experience for the viewers!

Fig. 1. In learning how to manage this display, I discovered to what degree olfaction is involved in robbing behavior. (We also have two free-flying observation hives in another booth, with the bees exiting via 1.5” pipes through the roof. The bees flying overhead do not cause any problems with the fairgoers.) Photo credit Gilles Ratia.
For our initial setup, we placed a hive in the cage a few days before the fair opened. The bees quickly learned to exit and enter through the open top of the enclosure, and there were no bees to be seen outside the cage where the viewers would be standing. All good!
When I gave my first demo, I stuck my finger into some recently-capped honey to show how we beekeepers enjoy tasting honey direct from the comb. To my surprise, within minutes a number of bees showed up on the outside of the screen directly in front of the entrance of the demo hive (which was a couple of feet back). So on subsequent demos, I was careful not to break open any cells of honey, and saw that bees didn’t suddenly show up again. So I started paying attention.
Over the years, we continued to be annoyed by small numbers of bees that would show up around the screen in front of the hive entrance. Trapping them didn’t help. It took some experimentation, but I finally figured out what was happening, and how to solve the problem.
Our Fair is held in August, when we are normally in a nectar dearth. What appeared to be happening was that the bees outside the screen were scouts from other colonies outside the fairgrounds, orienting to the odors in the air exhausting from the hive entrance inside the enclosure.
So how to move the hive entrance? It took a bit of experimentation to determine that foragers can easily circle up and down within a column of Langstroth hive bodies. This allowed me to route bee flight (and ventilation air) from the demo hive through a stacked column of empty supers going up to the top of the cage (10 feet off the ground). Problem solved — no more curious bees on the screen! It was clearly the odor of the hive exhaust that had been attracting them.
We continue to experiment, and last summer replaced the upper boxes with a chase of clear plastic, so the audience can watch the bees fly up and down (Figure 2).

Fig. 2. My helper Rose Pasetes (dressed for enjoying the fair with her husband) dropped in to join me for this demo. You can see the clear plastic flight column behind her head.
I’m well aware of how incredibly sensitive honey bees are to odor, and how easily they can be trained to associate an odor with a sugar reward (in the field, such as how Jerry Bromenshenk trained bees to locate land mines, or in the lab via the “proboscis extension response” (PER) [[1]]). So after observing the screen-thwarted robbing pressure on our demo hive, I’ve paid close attention to robbing behavior in our apiaries and around our honey house. I’ve noticed a number of things:
- We can have stacks of unused brood chambers containing honey sitting on pallets in the yard, and bees may not pay any attention to them for weeks or months, unless we break open the honey.
- When we’re extracting honey, the vent of the extracting room will be mobbed by bees if we’re extracting a honey that was recently gathered, but otherwise there is far less interest.
- Ditto in our storage yard: if a scout happens to come across some exposed honey, they will quickly return with recruits — and seek out any other honey of the same floral variety stored in the yard.
- When removing honey supers, robbing is far more intense if there is still ripening nectar in the hives than it is if we wait until that specific nectar source has not been being gathered for a while. Potential robbers appear to cue in on the specific odor of ripening nectar from whatever flower species they are currently or had recently been working.
- Our nucleus colonies can get the heck robbed out of them while there’s a strong nectar flow going on.We’ve seen this happen to an entire yard of nucs – leaving them cleaned out of honey by the end of a strong springtime flow, after watching heavy robbing clearly taking place during morning foraging with little or no fighting at the entrances. The foragers of the nucs appeared to just continue with normal foraging, while robbers from somewhere else went in an out without interference.
- When we have a nectar flow on, robbers quickly descend upon combs of ripening nectar if we pull them from hives.But at the same time in the same yard, where we have returned hive bodies containing plenty of combs with patches of sealed honey, stacked wide open for robbing, the abundant bees show no interest.
It’s pretty clear that scouts and foragers are exquisitely sensitive to (and cue into) specific scents with which they’ve learned to associate with a sweet reward. On the other hand, they may ignore the scent of exposed honey to which they have not yet associated with a reward.
Practical application: All one need to do to demonstrate how closely tied odor is to robbing, is to feed sugar syrup with added scent to a group of colonies during a dearth.
BACK TO WHAT HAPPENS UPON COLONY COLLAPSE
Where Peck and Seeley ran their study there is a mid-September nectar flow in Ithaca, NY) [[2]], with a distinctive goldenrod odor. Since their mite-donor colonies collapsed in late-September, they would likely have been emitting the odor of ripening goldenrod nectar. That would have made them very attractive to scout bees from other hives searching for any source of that odor (since the bloom would have been tapering off, and the scouts and foragers would have already been trained to associate that specific scent with a sweet reward). Thus we would expect immediate robbing pressure on any collapsing colonies to take place.
On the other hand, where I live, the typical observation is that “my colony was strong in August, and then the bees suddenly disappeared, leaving a hive full of honey, but without any bees.” I’ve seen it plenty of times myself, and heard it over and over again from those coming to purchase replacement nucs the next year.
Practical application: Our collapsing hives don’t contain any ripening nectar, so even a handful of bees are able to defend them. And even after those last bees are gone, the deadout often sits for days or weeks before it eventually gets robbed. If the bees are all gone by the time robbing takes place, then there wouldn’t be any live mites left to transfer onto the robbers (any mites still in remaining sealed brood would be unable to escape through the cappings.).
So the question to me was, does such lack of robbing during collapse occur elsewhere. So I asked some other beekeepers around the world.
A SURVEY ON WHETHER ROBBING TAKES PLACE AT COLLAPSE
I put out a request to members of the Bee-L discussion group to fill out a survey. I asked them the following questions:
- Your region? (state, country, province, etc).
- The typical period of varroa/DWV collapses in your region? (month – month).
- Do varroa/DWV collapses in your area typically occur during or immediately after a nectar flow, or during a dearth? (during flow, immediately after, dearth)
- Do the collapsed hives get robbed out immediately or later? (immediately or later).
Fifty-one beekeepers responded; their answers are in Table 1 (names redacted).
Table 1. Survey on observations of colony collapse and robbing. Note how many respondents stated that robbing occurs later, rather than during collapse.

I noticed that Dr. Peck’s published paper [[3]] included the following:
Because it has been observed that bees in some locations do not readily rob weak colonies in the autumn (Randy Oliver, pers. comm.), it is entirely possible that the robbing-driven “mite bomb” phenomenon that we observed in New York state may differ from the “mite bomb” phenomenon in other places, where drifting may play a larger role, or robbing a smaller one.
MY CONCLUSIONS FROM THE SURVEY RESPONSES
Robbing of collapsing (as opposed to collapsed) hives can clearly result in mite transfer from the hive being robbed to the robbing hive, but only in those areas in which robbing occurs at the time of collapse.
- Colonies kept in areas with long winters collapse later in the season—likely because varroa buildup starts later.These colonies often collapse when the weather is below flight temperature, so no robbing or mite drift can take place.
- In Mediterranean climates with summer dearths, collapse appears to seldom be associated with robbing.I suspect that this is due to scouts or robbers in the neighborhood not associating the slight scent of the well-cured honey in those collapsing hives with a reward.
- An exception to the above is where Africanized bees are established, such as in the southern U.S. (including Southern California) where the robbing pressure by AHB can be intense.
- On the other hand, in areas where there is a nectar flow occurring or tapering off during the collapse of a colony, those hives can face immediate exploitation by robbers during their process of collapse. I suspect that this is due to scouts, foragers, and robbers in the neighborhood having learned to associate the scent of that ripening nectar with a reward.
RELATIONSHIP TO BEE AND MITE DRIFT
So in Mediterranean regions, there may not be much mite immigration due to rob outs of collapsing colonies. But that still leaves the question of what happens to all the bees and mites in those collapsing hives? (They may vanish, but not into thin air). Other studies indicate that they don’t appear to show up in nearby colonies to any great extent. Follow the results of my field trial with tagged bees to see what occurred at my neighborhood.
A HUMOROUS ANECDOTE REGARDING TRAINING BEES TO A SCENT
Years ago I pulled into an outyard during our August dearth, and noticed a scrawled handwritten note on top of a hive. A neighbor had written that he had been attacked in his driveway by our bees!
Incredulous, I drove over to see what happened. He sheepishly apologized, since he had since realized that he hadn’t actually been attacked. Then he explained what had happened.
He told me that he was a Dr. Pepper “junkie,” drinking a few cans a day, and threw the empty cans into a recycling can in his garage. On the day of the “event” he had accidentally left the door open, and returned to a garage full of hungry bees scavenging the remaining sugary residues in the cans.
Come dusk, after the bees had gone home, he closed the garage door.
By noon of the next day there were no more bees to be seen around the garage. Proud of his victory, while standing in the driveway he opened a can of Dr. Pepper to celebrate. Unnoticed foragers still searching for the scent of the previous day’s prize immediately descended upon the can in his hand. Luckily he didn’t do anything foolish that might have led to a sting.
I commended him for having trained the bees to the scent of Dr. Pepper, gave him a case of honey, and all was well.
ACKNOWLEDGEMENTS
I want to honor my late friend Tim Madeira, with whom I built our demo cage, shortly before he unexpectedly passed away. Appreciation to Drs. David Peck and Tom Seeley. And thanks to those who filled out my survey. Dr. Peppers all around!
CITATIONS AND NOTES
[1] Smith, B & C Burden (2014). A proboscis extension response protocol for investigating behavioral plasticity in insects: application to basic, biomedical, and agricultural research. Journal of Visualized Experiments (91): e51057.
[2] Seeley, TD (1995) The Wisdom of the Hive, p. 44.
[3] Peck DT & TD Seeley (2019) Mite bombs or robber lures? The roles of drifting and robbing in Varroa destructortransmission from collapsing honey bee colonies to their neighbors. PLoS ONE 14(6): e0218392.
About me
To start with, among other things, I’m a honey bee researcher, so if you’re not comfortable with honey bees being around, read no more
I’m a healthy, youthful, happy, high-energy, and loving guy, finally over the heartache of losing my beloved wife. So I’m now looking for a new life partner and soul mate with whom to share my exciting, productive, and joyful days.
I am a biologist, nature lover, family man, teacher, inventor, writer, gardener, and builder. My friends would describe me as the busiest guy they know, and the guy who gets things done. The answer man to any question. Playful and funny. My joy is sharing with others – I’ve always been a teacher and giver. Helpful, loving, sharing. Widely loved by many.
I maintain amicable relationships with those with whom I’ve been in previous relationships. They, and the women that I work with, will all vouch for my loving good nature, honesty, and trustworthiness. And if you’re familiar with the five love languages, mine is clearly touch — hugs and intimacy warm my soul.
Disclaimer: My eharmony photo is from 2018 , but it best captures my essence. Here are some more from this year.
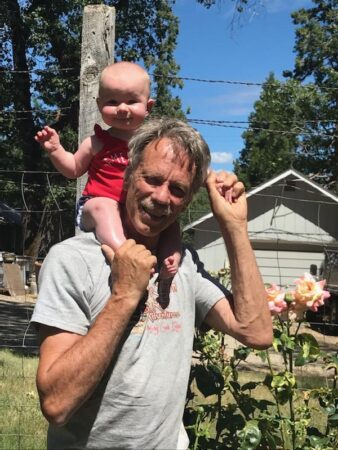
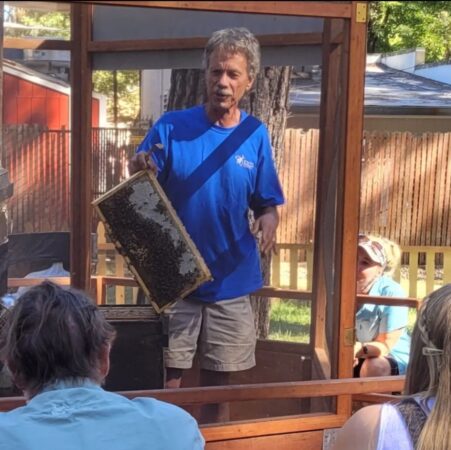

Possible Incompatibilities
Since I’m not in any way trying to seduce you (and have no desire to waste our time), please let me share some things about me and my lifestyle that might suggest incompatibility:
- I’m a honey bee researcher (writer and international invited speaker). You’d need to be OK with being around honey bees.
- If you’re looking for a quiet life in front of the TV, read no more. I’m a high-energy, high IQ, vibrant, scientifically-minded guy, biologically about 10 years younger than my chronological age, lean and very active, and I work nonstop — so am not the right partner for a low self-esteem or low-energy lady (although I’m an intense and focused hard worker, I’m also very happy and humorous, always smiling, laughing, and joking).
- I’ve got many skills. And just like the cobbler who can’t find time to shoe his children, my houses are in various stages of remodeling and disarray.
- Although I’m financially set up, I live a simple, rustic, eco-friendly lifestyle, and wear my jeans for more than one day at a time.
- I am looking for someone who wants to move to my place. I’ve got a rural property in the California foothills (Grass Valley), where we get a bit of snow in the winter. I grow a large garden and orchard, and am looking for a partner who would enjoy being involved and engaged in the garden and the property (woodsy with a lovely view).
- I’m more of a cat person than a dog person. I like the wildlife around my home, and don’t want a dog scaring everything off.
- I’m not the right person for someone who is intimidated by intelligence, or put off by me being so engaged in my research, writing, shop work, and property maintenance. I can also be kinda messy, since I always have so many projects in the works.
- As far as a partner, I’d prefer an energetic, physically active, preferably lean, and affectionate woman who enjoys a simple rustic, eco-friendly lifestyle. I follow the Golden Rule, but have no interest in organized religion.
More about me
I’m likeable, happy, and appreciated by others. I’ve got no issues or traumas, and am responsible and caring. I exemplify the Scout Laws (I’m the inverse of our ex-president). You will never hear an untruth cross my lips.
I’m an ex hippy child of the ’60s, now a responsible, environmental, fiscally-conservative, socially-liberal adult. I was raised to honor and respect women, and have no desire to dominate a relationship.
I’ve got many skills, and am highly competent, capable, and accomplished. I’m always happy, free of stress, loving, generous, and looking for a soul mate and partner to share the everyday joys of life with — like a new flower blooming, or the taste of the first fruits from my orchard, or a beautiful sunset.
I live on a dream property with garden, orchard, and a view, am in good health, and financially stable. I’ve got two houses next to each other (one vacant since my mother passed away), and I’d be happy to remodel either one to my new partner’s likings.
I’ve handed my sons our beekeeping business, so am now free to follow my passion of performing honey bee research and providing scientific information to beekeepers worldwide. My sons run the business from my property (one lives next door), so we often have visitors and things going on at the property.
I lead a simple, frugal, rustic life, in an older house – nothing fancy (other than traveling to speak). I’m early to bed, early to rise. In my office before sunrise, cranking data, writing, email correspondence, and reading scientific papers. After breakfast, in and out for the rest of the day. Other than the news, I watch very little TV. Back in for dinner, which I enjoy cooking. I love spending evenings watching a movie together with the one I love.
I’m home or nearby most days, and don’t go to town much, but do travel to speaking engagements, which I try to limit to no more than once a month.
I respect and honor women, and have worked with ladies most of my life. I’m well liked and loved – any of my ex’s or female coworkers will give me a thumbs up.
The partner I’m looking for
My previous relationships have been based upon love, attractiveness, and intimacy, but after signing up for eharmony, I realize that I’d be wise to find someone whose energy and personality traits match mine. I’m a likeable guy, so I’m not desperate or trying to sell myself, seduce or mislead you, so I will be honest and straightforward. I do not want to waste your time or mine.
As far as relationship, since I’m a brainy, strong guy, I want an equally strong, self-assured partner who can stand up for herself, and communicate and articulate her feelings and wants. I don’t argue or criticize, and have zero desire to dominate or always get my way.
Although I very much love doing things together, I need a partner who also has her own interests and hobbies to happily entertain herself while I’m working on my own projects. I’m far more interested in an active tomboy “country girl” in dirty jeans than a beauty queen in high heels. I want a gal who doesn’t mind getting dirt on her hands, and will enjoy working in the garden and orchard with me, and be my third hand when I’m working on projects (and ditto for me helping with yours).
I ain’t perfect, and don’t expect you to be either. I want my partner to accept me in full as I am, and I will do the same in return. I don’t want to merely love my partner, I want to be in love with her every moment of my life!
Contents
Extended-release thymol 1
The optimal release rate and placement of thymol 1
What’s The Optimal Dose?. 2
Our Field Testing. 3
The Dose-Response Curve. 12
Discussion. 12
Citations and notes 13
Thymol — A new application method?
Part 2
First published in ABJ in December 2022
Randy Oliver
ScientificBeekeeping.com
I do a lot of experimentation in beekeeping, often triggered by questions or ideas from other beekeepers. This spring a beekeeper showed me a homemade thymol concoction, which reminded me to pick back up on some previous thymol research of my own.
Extended-release thymol
Last month I raised the question of whether we could use a ½” thick cellulose matrix (Homasote [[1]]) in order to throttle the evaporation rate of a thymol treatment over a longer period of time, thus allowing us obtain efficacious mite reduction with only a single application.
A scientific note: Before investing time in large-scale controlled trials, I generally first perform some preliminary “quick and dirty” experimentation, as detailed here [[2]]. Not needing to follow a rigid protocol, this opens the door for ad hoc experimentation and unanticipated discoveries, which may then lead me in new directions.
The optimal release rate and placement of thymol
I’ve mentioned that bees don’t care for the odor of thymol, and if a treatment is placed above the cluster, they may build a wall of propolis around it (Figure 1).
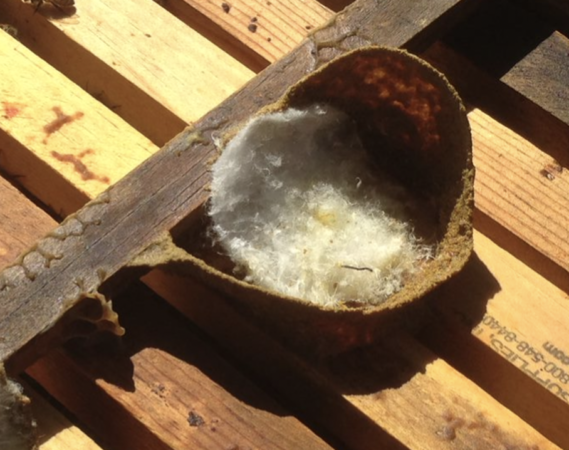
Fig. 1. If the bees are unable to remove a substrate with a strong odor of propolis, they will attempt to cover or wall it off with a layer of propolis. Of interest, I did not observe any colony doing this with the Homasote blocks.
Practical note: A strong thymol treatment applied within the cluster can be very disruptive to the colony, causing the bees to noisily attempt to fan the odor away, uncap and remove brood (with lots of white pupae showing up on the bottom board), or in the worst case, the colony may completely abscond from the hive.
I found that it’s not just the dose, but rather the amount of surface area from which the thymol (with its low vapor pressure) is able to evaporate. Thus, application by thymol-saturated shop towels, with their large surface area relative to volume, results in too rapid release of the thymol (especially when applied between the brood chambers, due to the bees intensely fanning air over the towel surfaces).
Thymol on a shop towel would be a “flash” treatment. On the other hand, the registered thymol formulated products apply thymol in an extended-release manner.
Practical application: But even those products require at least two applications. So I wondered whether in order to save the labor involved in reapplication, is there a way to prolong the release of a larger dose?
What’s The Optimal Dose?
Thymol can be applied as a flash treatment to broodless colonies, with most mites dropping within a few hours of application. Chiesa [[3]] sprinkled 1 gram of powdered thymol over the tops bars of broodless colonies, four times, at 2-day intervals, and obtained over 95% mite kill, especially if the powdered thymol was mixed with powdered sugar. So 4 g of thymol appears to be enough to kill the mites in a colony not containing brood.
But when colonies contain brood, it apparently takes a larger dose, delivered over a period of weeks rather than days. Let’s take a look at the number of grams of thymol per dose of the registered products (the red numbers in Table 1).
Table 1. The per-application dose of registered thymol formulated products. |
Product name |
Dose of thymol per application |
Retreatment interval and number of applications |
Delivery matrix |
Other ingredients |
Apiguard |
12.5g for full-strength colonies, half that when temperature is above 77°F |
Repeat at 1-2 week intervals for 4-6 weeks |
Polyacrylate “gel” in water |
None |
ApiLifeVar |
8g |
Repeat 3x
(some instructions say 4x) at 7-10 days |
Vermiculite |
Eucalyptol, menthol, and camphor |
Thymovar |
15g can be ramped up in hot weather |
Remove after 3-4 weeks, pause for 1 week, then reapply |
Cellulose sponge |
None |
It looks as though a colony with brood can handle 6-12 grams of slow-release thymol per week. Since I’d already determined that 5.1 square inches of Homasote will hold 12 g of thymol (after absorbing a saturated solution of thymol in ethanol), it was a simple matter to precut blocks of Homasote to hold any dose of thymol that I wanted to test. I decided to apply four blocks of Homasote per hive, so I calculated how large to cut each block to attain each desired dose per hive (Table 2).
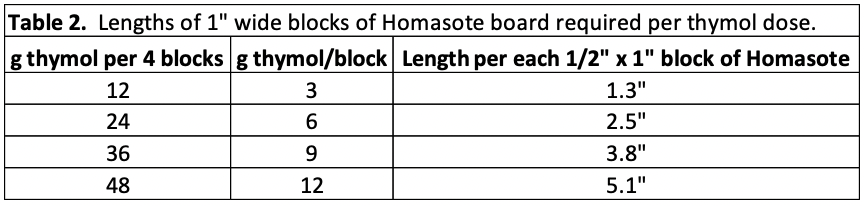
Our Field Testing
It was late August when we started this project, so unfortunately when I asked my sons as to the availability of colonies with high mite counts in which I could test these experimental thymol treatments, they said that all their 1500 colonies had the mites well under control, with mite wash counts (per half cup of bees) in the 0-2 range. This was good for them, but bad for me.
So the only colonies with high enough mite levels to use for testing were the hives left in three yards in which Rose and I had previously tested formic and oxalic treatments, some of which still had measureable mite infestations. So we started testing to see how the colonies reacted, in hot weather, to having a 12-gram dose of thymol placed right between the brood chambers (Figure 2).
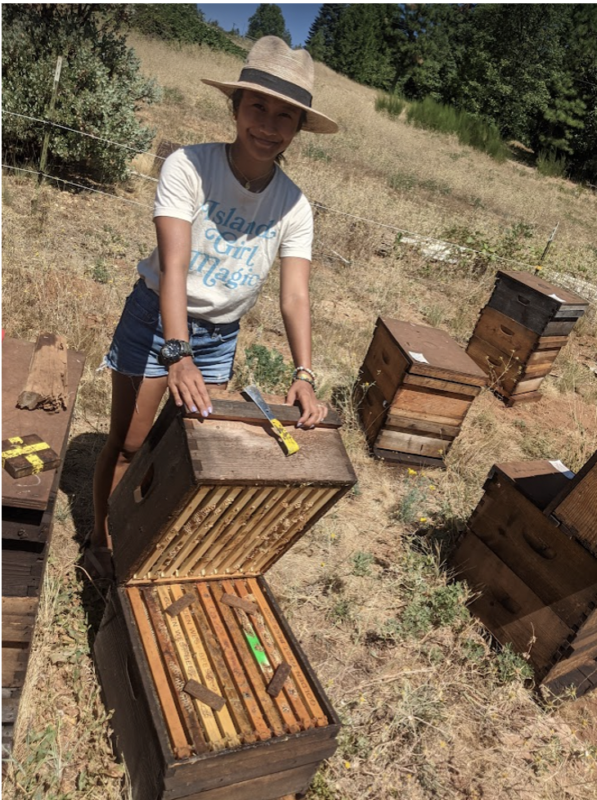
Fig. 2. My assistant Rose Pasetes (wearing proper protective gear against the sun) showing the placement of four blocks (this photo is of a moderate dose). The bees initially moved away from the fumes, so it’s not obvious in this photo that the hive was full of bees and brood.
We applied the 12-g dose to six hives. To our great surprise, within a day the bees acted as though the thymol wasn’t even there. We could smell the fumes when we opened the hives, but there was no fanning, and no apparent effect upon broodrearing (Figure 3).
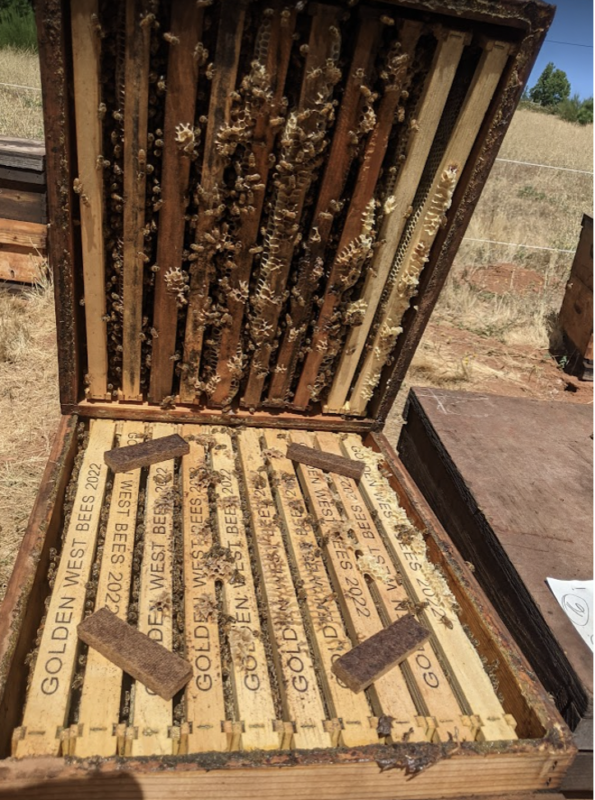
Fig. 3. Again, a higher dose, but showing the lack of disturbance to the bees in this freshly-opened hive.
Surprised and emboldened by the lack of bee response to the 12-g dose, we applied 24-g doses to seven colonies, some with the blocks applied between the brood chambers, some in a rim above the second brood chamber (Figure 4).

Fig. 4. Typical placement of thymol blocks in a rim.
I opened the colonies regularly to inspect for the sort of brood disruption I was accustomed to seeing during a thymol treatment, but even with the 24-gram dose I didn’t see anywhere as much as I expected. So what the heck — we made up some more blocks in order to apply 36-g doses to seven additional colonies.
During this ongoing experimentation, I was able to observe what the bees did as the intensity of the thymol fumes wore off in the first hives. As I’d noted in my experiments in 2017, most colonies began chewing away at the blocks, rather than propolizing them over (Figures 5 & 6).
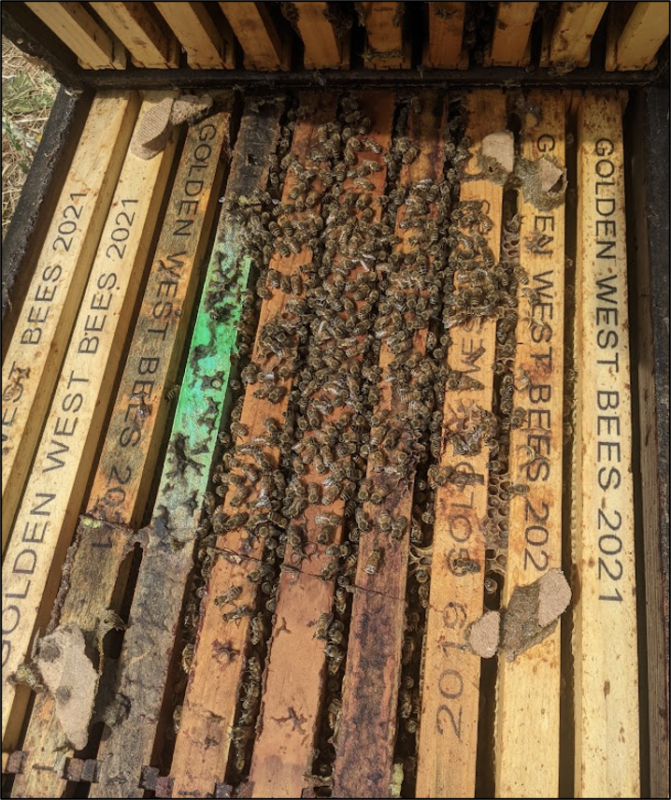
Fig. 5. The blocks still smell of thymol after three weeks, but the bees don’t appear to be bothered by it by that time. Once the thymol fume intensity diminished, the bees in most of the hives started chewing away at the blocks. There were of course large colony-to-colony differences in the amounts of propolization and chewing removal, but this photo is typical.
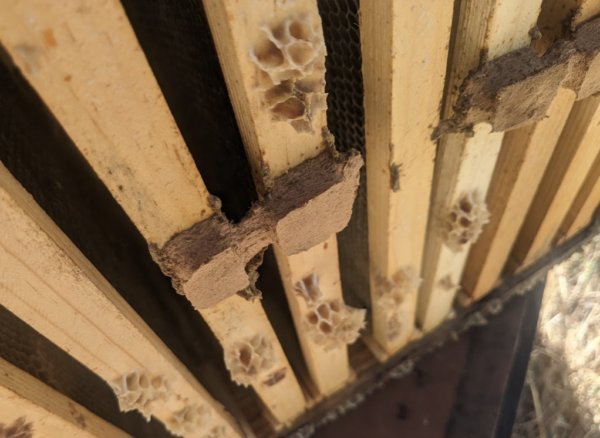
Fig. 6. Note how where evaporation was inhibited by the blocks being squeezed between the top and bottom bars, that there was chewing only on the edges.
What surprised me most about this experiment was how little the colonies appeared to be bothered by these high-dose treatments. There was no noticeable fanning, and after an initial suppression of the rearing of young larvae, most colonies quickly resumed the rearing of all stages of brood in the center frames — no matter whether the blocks were placed between or above the brood chambers.
I was even bold enough to apply 48 grams of thymol, in a rim, applying the treatment to a strong double on 27 July, with temperatures often in the high 90sF (Figures 7 & 8).

Fig. 7. Photo on 17 August — 21 days after application of four 12-gram thymol blocks — of healthy brood of all ages in the lower brood chamber in the heat of summer drought. The solid sealed brood indicated that the larvae had been started and reared during the most intense period of thymol evaporation. I could hardly believe my eyes! The mite wash count had gone from 14 mites to zero.
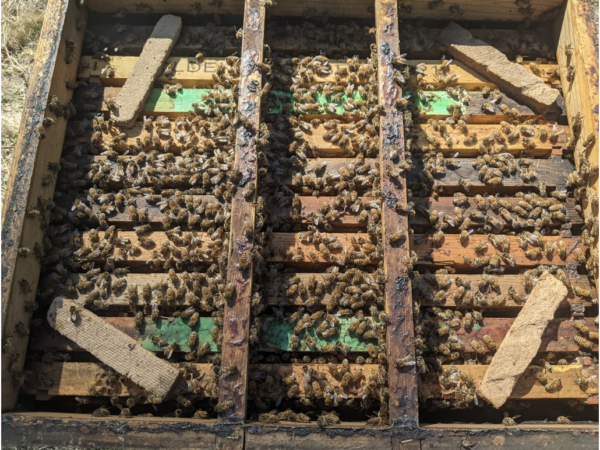
Fig. 8. Same colony on Oct 9, 74 days after application of blocks (total 48g of thymol), which by then had no noticeable thymol odor. The mite wash count was still zero, with the colony containing plenty of good-looking brood and honey.
Surprised and emboldened by how well this colony did with 48 grams of thymol placed in a rim (and needing more data for a dose-response curve), in late September I identified two (unfortunately) weak colonies with measureable mite counts, and placed four 12-g blocks between the brood chambers (Figure 9). Weather was in the 70sF, but soon rose again to the 90s. Unfortunately the nectar and pollen flows were over, and there was little broodrearing taking place before or during this intense treatment, but I wanted to see (1) whether a 48-gram dose would zero out the mite counts as it did with the colony above and (2) to see how weak colonies would respond to this extremely high dosage of thymol.
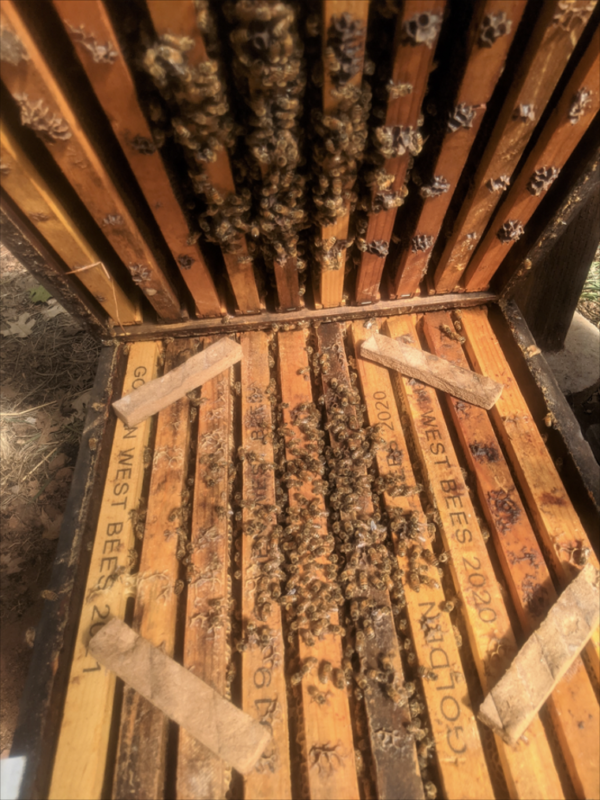
Fig. 9. A weak colony four days after application of four 12-g thymol blocks late in the season (an intentionally intense test). Due to dearth there wasn’t much brood when I applied the blocks, but even after four days of this intense exposure, there were still patches of brood of all ages on three frames in the upper box. Other than their immediate buzz when I had first applied the strips, the bees did not appear to be disrupted by the obvious odor of thymol – the bees appeared to be acting “normally.”
I’m not about to say that there was no adverse effect from this strong dose upon the colonies, but I was surprised by how the bees appeared to quickly acclimate to the odor of thymol in the hive (it was easy for me to smell when I opened them). These two weak 48-gram colonies continued to rear some brood of all ages throughout the treatment, and their mite wash counts had dropped to zero by 17 days after application (one going from a count of 44 mites to zero).
The Dose-Response Curve
I was only able to find 23 colonies with mite counts high enough on which to test this method of thymol application (having starting mite wash counts ranging from 5 to 44; mean 20, median 18), so this data is only preliminary and suggestive. In order to estimate a dose-response curve, I calculated percent reduction of the counts vs. the applied dose of thymol, so that I could get an idea as to the optimal dose to test next season (Figure 10).
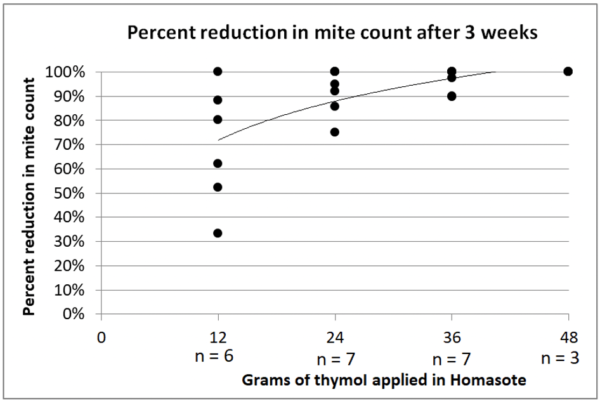
Fig. 10. The higher the data points, the better the mite reduction — 100% indicates and ending count of zero. A 12-gram dose (divided between 4 blocks) gave inconsistent mite reduction, but 48 grams zeroed the counts in all three hives. The less-intense 36-gram dose may be the optimal target dose, but it’s not yet clear whether it is best applied between the brood chambers or in a rim above (I tested both).
Discussion
As a long-time user of Apiguard, I’m pretty stoked by the convenience of this application method of thymol in Homasote blocks. I was dumbfounded by how gentle the 12- and 24-gram doses applied in this manner were on the bees and brood, and pleasantly surprised by how well the colonies handled the 36- and 48-gram doses. I plan on obtaining a permit to expand my testing of this method next season.
Practical application: An easy-to-apply thymol treatment that requires only a single application would be great to use in rotation with oxalic and formic acid.
Note: This application method is not approved in the U.S, but could be used by beekeepers in New Zealand or other countries where it is legal.
Addition: Checking the Safety Data Sheet for Homasote, I find that it contains a tenth of a percent (1000 ppm) copper metaborate (likely to inhibit mold and termites).

So this raises the question as to whether that amount of copper metaborate is of concern as far as adverse effects upon the bees, or contamination of honey (unlikely, since we don’t normally apply thymol treatments during a honey flow).
The EPA concluded in 2009 that “Available data from a honey bee acute toxicity study indicated that copper is practically nontoxic to honey bees.” (https://www3.epa.gov/pesticides/chem_search/reg_actions/reregistration/red_G-26_26-May-09.pdf), apparently based upon a single study:
Hoxter, K. (1988) Copper Hydroxide: An Acute Contact Toxicity Study with the Honey Bee: Wildlife International Ltd. Project No. 190- 140. Unpublished study prepared by Wildlife International Ltd. 14 p.
A point to keep in mind is that it’s unlikely that a bee would consume any of the Homasote. I did not observe any indication of adverse effects after treatment, but will run some cage trials.
Hladun, K.R., Di, N., Liu, T.-X. and Trumble, J.T. (2016), Metal contaminant accumulation in the hive: Consequences for whole-colony health and brood production in the honey bee (Apis mellifera L.). Environ Toxicol Chem, 35: 322-329. https://doi.org/10.1002/etc.3273
Citations and notes
[1] http://www.homasote.com/assets/files/homasote-440-sds.pdf
[2] For this off-label experimentation with thymol, I obtained a Pesticide Research Authorization from the California Department of Pesticide Regulation, since unlike with my previous research that did not involve using thymol as a miticide, in this case I was using it for that purpose.
[3] Chiesa, F & M D’agaro (1991) Effective control of varroatosis using powdered thymol. Apidologie 22(2): 135-145.
Contents
“Off-Label” use of thymol 4
Thymol products for varroa control 5
Understanding Thymol 6
Reaction to the delivery matrix by the bees 6
Thymol in Shop Towels. 7
Removal and dispersion of thymol matrices by the Bees 9
Extended-Release Thymol?. 11
A Thicker Matrix. 11
moving ahead to 2022. 15
Citations and notes 15
Thymol — a New Application Method?
Part 1
First published in ABJ in November 2022
Randy Oliver
ScientificBeekeeping.com
As our summers get hotter, some of the “natural” treatments against varroa have issues, since the adverse effects of both formic acid and thymol increase at high temperatures. Thus I’ve been experimenting with reducing and extending the release rate of oxalic acid and formic acid. But how about thymol — could we extend its release rate too? This summer I resumed some research that I’d initiated back in 2017.
Thymol is a well-proven “natural” miticide, fairly well tolerated by the bees. My sons and I use thymol in rotation with oxalic acid and formic acid, with each miticide being appropriate for use during different “treatment windows” (Figure 1).

Fig. 1. I created the above generic chart (adjust the dates for your area) to point out the best treatment windows for varroa management, as well as the most appropriate active ingredients to apply during those windows. We’ve found that in the California climate, it typically requires at least four treatments per season to maintain low varroa levels (in non-resistant stock). To avoid the evolution of miticide-resistant varroa, we all must rotate different active ingredients.
Thymol, due to its temporary suppression of broodrearing, is appropriate for use if you can get harvestable honey off the hive before mid-August. It can also be used to both control mites and suppress colony buildup prior to swarm season.
In my state of California, there is only a single registered thymol product — Apiguard, with specific instructions as to how it can be applied. We’ve been happy with Apiguard, but that’s not to say that there might not be a more bee- and beekeeper-friendly way to apply this proven miticide. Some limitations of Apiguard are that:
- A space must be provided below the hive cover, which means that for those of us who use migratory covers, a spacer rim of some sort must be provided (Figure 2).
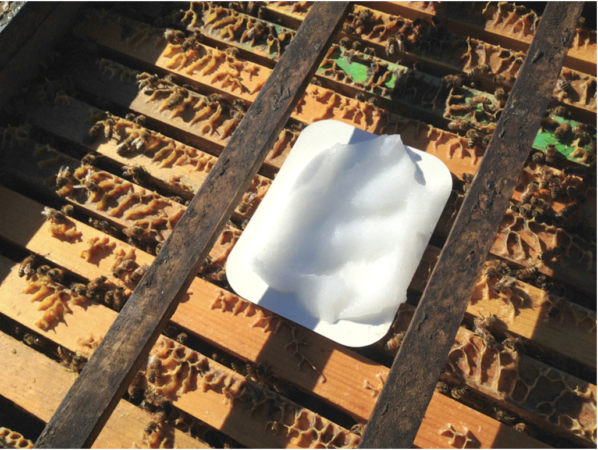
Fig. 2. The only thymol product approved for use in California is Apiguard “gel,” here applied in a spacer rim. My sons and I have successfully used it for many years in rotation with other treatments, mainly during hot weather in August, when we don’t mind its temporary suppression of broodrearing during our dearth period.
- For full efficacy, the treatment must be repeated, which takes extra labor. We’d much prefer a treatment that requires only a single application.
- The label no longer allows for placing the product between the brood chambers, which would be important for application during cool weather.
- Application above the brood chamber is only efficacious if the colony is strong enough to remove the treatment from the card above the top bars (my own observation). A treatment that could be applied between the brood chambers would be of great benefit.
- Although Apiguard can be applied at daily highs of up to 105°F, our summers are getting warmer (Figure 3), and during our optimal treatment window in August this year, it got even hotter than that.

Fig. 3. The blue trendline reflects the increase in California’s average August high temperature over my lifetime. It’s not just that I’m getting older — it really is getting hotter! You can plot similar charts for your area at https://www.ncei.noaa.gov/access/monitoring/climate-at-a-glance/statewide/time-series.
- Perhaps most importantly, when we apply Apiguard as per the label, the broodnest is greatly impacted — the vapor kills the eggs and young larvae directly below the treatment (which the nurses then cannibalize), and then the colony reestablishes a divided and reduced broodnest to either side of the treatment.
Practical application: Apiguard is a great product, but I suspect that there could be even better application methods appropriate for our conditions and colony management. But we beekeepers are legally precluded from tweaking the application methods specified on the label, or from using generic thymol to apply by different methods.
“Off-Label” use of thymol
Practical application: Varroa treatments are registered as pesticides. When a manufacturer goes through the tortuous and expensive process of registering a pesticide, they must provide a specific set of approved application instructions. Any deviation from those instructions is a violation of the law.
The situation is more reasonable with medicines: “From the FDA perspective, once the FDA approves a drug, healthcare providers generally may prescribe the drug for an unapproved use when they judge that it is medically appropriate for their patient” [[1]]. This is referred to as “off label” use, and is very common in medicine.
Doctors and their patients are allowed to experiment and learn about how best to use medicinal drugs under different circumstances, and for not-yet-registered uses. Unfortunately, this freedom does not apply to registered miticides, so we beekeepers (or even our veterinarians) are not allowed to tweak application methods to account for the wide range of situations that we encounter in beekeeping.
EPA’s refusal to consider granting us beekeepers an Own Use Exemption for the organic acids and thymol frustrates me, since it prevents us from learning how to apply these safe and inexpensive generic substances to our colonies, instead forcing us to purchase and follow the rigid label instructions for expensive registered formulated products.
Here’s the rub: As explained by USDA [[2]]: “Relative to miticide uses on agricultural crops, applications of varroacides to bee colonies are considered a minor use that may not allow for registrants to justify the costs to develop varroacides and break-even on such investments, given the small relative market potential.” So we can’t expect manufacturers to bring a bunch of customized varroa control products to market.
A plea to our national organizations: Registrants are reluctant to go through the tedious process of updating their labels, so we beekeepers are stuck between a rock and a hard place. As far as EPA’s mandate to avoid unreasonable risk to man or the environment, beekeeper use of generic off-the-shelf formic, oxalic, or thymol would pose no such risks. The government of New Zealand wisely recognized this, and gave beekeepers an exemption to use these “natural” products for varroa control however they wish. I suggest that our national organizations approach our senators and congressional representatives to legislate an Own Use Exemption similar to New Zealand’s.
The reality is that due to our limited arsenal of approved formulated varroa-control products, many beekeepers have unfortunately become “pesticide scofflaws.” I gotta walk a fine line with my research, which I do for the benefit of all, rather than for profit. I obtain Pesticide Research Authorizations from my State’s department of pesticide regulation, and publish my findings as scientific research only — I am not in any way suggesting that beekeepers use any unregistered product, or not follow label instructions exactly (I gotta cover my donkey).
Thymol products for varroa control
There are three main thymol products registered in North America for varroa control — Apiguard, ApiLife Var, and Thymovar. Only two are registered in the U.S., and only Apiguard in California. All work by the evaporation of thymol vapors from an inert matrix, and call for placing the treatment a few inches from the brood. Thymol is generally recommended for application during moderate ambient temperatures — typically between 20-25°C (~68-77°F), although some product labels allow application at higher temperatures. All specify not to apply while honey for harvest is being produced.
One widely-used product is ApiLife Var, which is a mixture of thymol, eucalyptol, menthol, and camphor, impregnated into a vermiculite wafer. I tried ApiLife when it was first released in the U.S. (read my report at [[3]]), and found that it was more irritating to the bees than straight thymol, likely due to the additional essential oils. But it may be this additional irritation to the bees — and mites — that gives it greater efficacy at low temperatures, or promotes its distribution throughout the cluster.
Thymovar, also registered in a number of countries, is a cellulose sponge strip impregnated with straight thymol crystals.
Apiguard uses a gel-like matrix of water and polyacrylate (such as is used in diapers) to suspend fine crystals of thymol, which slows the evaporation rate. In my experience, its efficacy is dependent upon the bees physically carrying away the particles, rather than simple evaporation.
I’m also aware that a number of beekeepers mix up their own “homebrew” thymol formulations, sometimes with the addition of other essential oils. I’ve tested some of these homebrews (which can be efficacious), but am most interested in straight thymol.
Understanding Thymol
Thymol appears to affect the mites by exposure to its vapor. It not only causes direct toxicity, but may also irritate them, or perhaps affect their critical sense of smell. In addition, thymol vapors can cause bees to engage in more intense cleaning behavior, or even brood removal. But it’s clear that thymol has a far greater adverse effect upon varroa than it does upon the bees (especially adult workers, who “get used to it”).
Contrary to popular belief, thymol is not highly volatile — if you put a few thymol crystals out in a tray to evaporate, it takes many days for them to do so, even at broodnest temperature. It’s really difficult to find data on thymol’s vapor pressure, but it appears to be far less volatile than water, kerosene, or perhaps even vegetable oil. If I stick my nose into the mouth of a jar of thymol crystals I can smell a faint odor; if I do the same with a jar of menthol crystals, the odor is intense, and my eyes and lungs burn.
The slow evaporation of thymol is affected by temperature, as well as the crystals’ exposure to air flow (which can be notably increased by the fanning of the bees). With Apiguard, the thymol is ground into fine crystals, which are suspended in a mixture of water and absorbent globules of sodium polyacrylate — very little thymol actually dissolves into water. Thus, the bees must actually remove the globules of gel to expose the surfaces of the crystals to evaporation.
With Thymovar, the crystals are more exposed, and can evaporate more quickly (sometimes causing excessive brood issues in hot weather).
Practical application: I wondered whether there was another way to apply thymol, that extended its release rate to avoid the need to reapply the treatment, that did not encourage propolization, that did not require the addition of a rim, and possibly be a matrix that the bees would remove themselves after treatment (such as happened when I applied a low dose of Apiguard between the brood chambers on an index card [[4]]). Ideally, a perfect treatment would not be excessively irritating to the bees, and would not shut down broodrearing for an extended period.
Reaction to the delivery matrix by the bees
Bees are repelled by a strong odor of thymol, and will attempt to enclose it in a curtain of propolis. But once its outgassing slows, the workers acting as “housecleaner/janitors” will either encase it in propolis (Figure 4), or pick off pieces of the matrix and remove it from the hive (Figure 6).

Fig. 4. If the cleaner bees are unable to chew away a matrix that smells of thymol, they will either build a wall of propolis around it, or encase it in a layer of propolis as above.
Thymol in Shop Towels
One of the delivery matrices for thymol that I tried was the commercial beekeepers’ standby — the blue shop towel. I found that a saturated solution of thymol could be easily made by dissolving it in denatured alcohol (especially if the alcohol was warmed) (Figure 5).
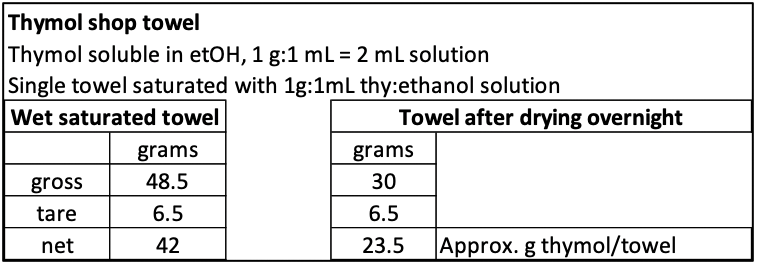
Fig. 5. A gram of thymol readily dissolves into a milliliter of denatured alcohol, resulting in ~2 mL of a saturated solution. The solution wicks readily into a shop towel. I then just laid the towels out to dry for an hour, by which time (at least in our California dry air) the alcohol had all evaporated. Due to the low vapor pressure of the thymol, there is nearly no weight loss of thymol. I then placed the dried towels into an airtight container for storage.
I applied the towels to test for removal and adverse effects. I placed half towels (containing a typical dose of ~12 g of thymol), in hot weather, either between the brood chambers, or in rims below the hive cover (Figure 6). It was quickly apparent that 12 grams on a towel in the middle of the cluster was too much, as the colonies roared and bearded out the entrances (I was afraid that they might abscond). That intense a dose also resulted in some removal of sealed brood. A quarter towel (containing ~6 g of thymol) was better tolerated.

Fig. 6. Half a thymol-impregnated shop towel in a rim. At first the bees are repelled by the fumes and fan them away. But as soon as the intensity of vapors drops, they rapidly remove every trace of the towel, rarely propolizing it.
What was interesting to me was the surprisingly rapid rate that the bees evaporated the thymol from the towels placed in the middle of the cluster. Unlike with Apiguard or Thymovar, it was like a “flash” treatment, and after a couple of days I could barely smell thymol in the remaining towels. Apparently, the temperature of the broodnest, coupled with the strong fanning, results in rapid thymol evaporation from this thin matrix, and janitor bees then quickly remove the remaining mildly scented towels.
A friend tried the towels in a few colonies, taking before and after mite counts. The results were that a half towel placed in the middle of the cluster resulted in substantial mite reduction, but was very stressful to the colony. A quarter towel was much better tolerated, but required a repeat application for efficacy. The towels placed in a rim above the hive gave variable results.
Practical application: The take home is that a shop towel, due to its high ratio of surface area relative to volume, allows for too-rapid release of the thymol for most purposes (but might be used in broodless colonies [[5]]). This thus raises the question of whether a cellulose matrix thicker than the Thymovar sponge would allow for a more controlled and extended release of thymol vapors as the thymol vapor from the interior of the matrix slowly diffused to the surface.
Removal and dispersion of thymol matrices by the Bees
Practical application: I’ve found that when Apiguard is placed above the colony, unless the bees physically remove Apiguard from the card it exhibits low efficacy against varroa. I suspect that the process of physical transport through the cluster is important, since it would expose the surfaces of the chewed particles of the matrix to allow for evaporation of the thymol. So an important question is, do the cleaner bees drag the particles down through the brood area (thus exposing the mites there to the fumes), or do they avoid that area?
We made an interesting observation some years ago when we had stickyboards under colonies treated with shop towels infused with oxalic acid and glycerin (Figure 7).
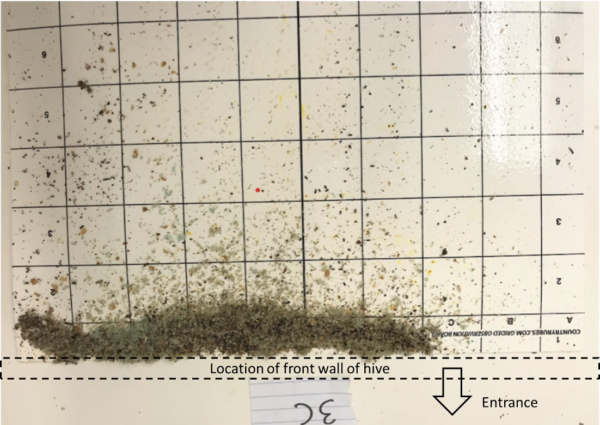
Fig. 7. When the housecleaner bees chewed away shop towels infused with oxalic acid and glycerin, the particles of debris nearly all wound up piled on the stickyboards, heaped just inside the entrance, suggesting that the janitor bees took routes that avoided the broodnest.
Curious, we recently set up a hive to determine whether the cleaner bees do the same with debris containing thymol. We put a stickyboard under a colony, and applied two different thymol treatments on the top bars between the brood chambers — one a half shop towel impregnated with thymol, the other scoops of Apiguard (mixed with a fluorescent tracer). We placed each treatment separately on different sides of the hive. I wanted to see whether the bees would again carry the debris to the front of the hive, or whether it would be taken (or fall) down through the broodnest (Figure 8).

Fig. 8. We placed fluorescent-tagged Apiguard on the top bars of one side of the broodnest (the far side in this photo), and a half thymol-infused shop towel on the near side. This image (under UV + natural lighting) was taken four days later, confirming that plenty of particles of each matrix somehow sifted their way directly down through the broodnest, rather than being carried out away from the brood (the gray-bluish debris in the foreground is from the shop towel). This dispersion of the matrix through the broodnest likely increases the exposure to the mites to the thymol vapors, since the thymol would evaporate rapidly from the surfaces of the small particles of debris as they were carried (or sifted their way) down.
Extended-Release Thymol?
Note that all the registered thymol products call for repeated applications to achieve good efficacy. If we could come up with a treatment that extended the release of thymol even longer than does Apiguard, we might be able to get by with only a single application.
This got me wondering whether we could use a thicker cellulose matrix than used by Thymovar, to throttle the evaporation rate over a longer period of time, allowing us to get by with a single application. And would a matrix that encouraged physical transport by the janitor bees increase efficacy?
A Thicker Matrix
I was intrigued by the Homasote insulation board (made from recycled newspaper) used by NOD to make its MiteAway II pads. So back in 2017, assisted by Tara McKinnon, we experimented with it. Other beekeepers were dissolving thymol in vegetable oil or Crisco for their homebrews, so I wondered whether the thymol would work better if canola oil was added to slow evaporation, or possibly help it to stick to the bees’ bodies. So we made up a 4-way grid of sixteen different thymol and canola oil concentrations (Figure 9), dissolved them in ethanol, and saturated 1” square blocks of Homasote with them (Figure 10).
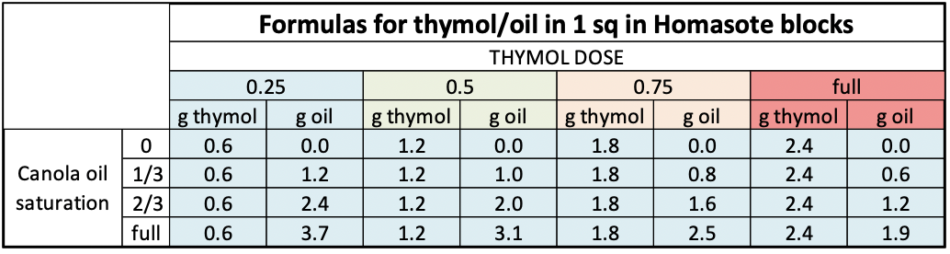
Fig. 9. We tried sixteen different ratios of thymol and canola oil. The amount of each substance that would remain in each 1” square block of Homasote is shown above. Those amounts ranged from 0.6-2.4 g of thymol, and zero to 1.9 g of oil.

Fig. 10. Preparing the test blocks. These have just absorbed the solutions.
We then placed sets of 16 test blocks into four hives between the brood chambers (Figure 11). This allowed us to test for how the bees responded to the intensity of thymol, as well as to the addition of oil.
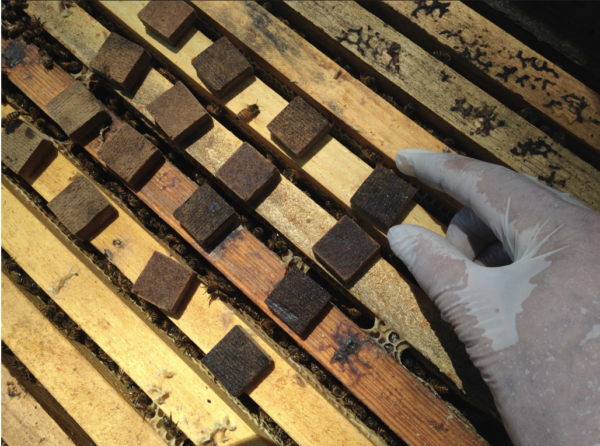
Fig. 11. Note the range of oil saturation, as indicated by the darker color of the blocks containing more oil. There were thymol crystals on the surfaces of all the blocks other than the most oil-saturated.
Tara then opened the hives daily, and recorded how the bees were responding to the blocks, as far as avoidance, chewing, or propolization (Figure 12).

Fig. 12. There was of course colony-to-colony variation, but we learned that the bees tended to propolize blocks containing more oil, whereas after a few days they started chewing away blocks with less oil.
This finding suggested that blocks of Homasote containing thymol alone (no oil), might work well as an extended-release cousin of the Thymovar strips.
moving ahead to 2022
I shelved the above thought until this year. Since I’ve recently been experimenting with extended-release formic and oxalic acids, I figured that I should pick back up on my experimentation with thymol and Homasote. But I’m out of space, so you’re going to have to wait until next month to see what we learned.
Citations and notes
[1] Understanding Unapproved Use of Approved Drugs “Off Label” | FDA
[2] Varroacide Registration : USDA ARS
[3] IPM 7 Fighting Varroa The Arsenal: “Natural” Treatments – Part 2 – Scientific Beekeeping The application instructions on the label are surprisingly confusing, but Veto Pharma has some useful videos on its website.
[4] The Learning Curve – Part 3: The Natural Miticides – Scientific Beekeeping
[5] Chiesa, F & M D’agaro (1991) Effective control of varroatosis using powdered thymol. Apidologie 22(2): 135-145.
Contents
Hard “Flash Treatment” vs Slow-Release. 1
Flash Treatments 1
Extended-Release Application. 2
our Follow-up experiments. 3
But Did the Wrapped Strips Kill Mites?. 8
Discussion. 9
Citations and Notes 9
Formic Pro in Hot Weather —
Slowing the Rate of Vapor Release
Randy Oliver
ScientificBeekeeping.com
First published in ABJ in October 2022
Last month I wrote about some “quick and dirty” preliminary experiments to investigate methods to possibly reduce queen turnover when applying Formic Pro during hot weather. We followed up with some more experimentation with the strips.
Hard “Flash Treatment” vs Slow-Release
The challenge when using formic acid for varroa control, especially in hot weather, is to find the “sweet spot” of enough vapor intensity and duration to kill the mites, without knocking out the queen.
Flash Treatments
A flash treatment is of short duration, measured in hours, as opposed to days. An overnight hard flash application of formic acid can indeed penetrate the cappings and kill the mites thereunder, but at the potential cost of causing the loss of the queen.
Dr. Jim Amrine wrote about using a hard “flash” treatment to control varroa [[1]], and my sons and I played with it a bit some years ago [[2]]. Amrine used 80-110 mL of a “weak” 50% formic acid solution, and obtained good kill of mites under the cappings.
Another flash treatment widely used by Canadian beekeepers, typically involves pouring 30-40 mL (sometimes more) of a more concentrated (60-65%) formic acid solution into meat pads (“mite wipes”), applying them on the top bars, and repeating three or more times, 7-10 days apart.
Practical application: The necessity of reapplying the lower dose of formic acid indicates a lack of efficacy at the killing the mites in the brood. There is a clear tradeoff between applying formic vapors strong enough for penetration and kill off the mites, but being “gentle” enough to not kill the brood and queen. The labor involved in the necessity of repeated applications led others to develop extended-release methods.
Extended-Release Application
Years ago, the Europeans, as well as others, developed extended-release methods to apply formic acid, involving placing an absorbent matrix saturated with formic into a perforated zipper-top bag or some sort of fancier plastic application device to regulate the rate of release.
Practical application: The evaporation rate of formic acid is dependent upon the exposed surface area of the delivery matrix, the temperature, and the humidity. Within the cluster, temperature and humidity are largely held fairly constant by the bees, but when an irritant such as formic acid is introduced, the bees may fan vigorously, causing more rapid ventilation, resulting in inadequate penetration of the vapors into the capped brood cells.
On the other hand, at lower concentrations of formic vapors, the bees quickly acclimate and ignore the fumes, and I suspect that they maintain near-normal temperature and humidity around the delivery matrix or device (if anyone has recorded data on this, I’d love to see it).
There are a number of adjustable formic application devices sold in Europe. The Nassenheider evaporator, invented by the German beekeeper Bruno Becker in the 1990s, is also widely used. It allows the user to precisely adjust the rate of formic vapor release. One suggested daily evaporation rate when using 60% formic acid is 15 – 20 mL in warm weather, or 6 – 10 mL when cooler. If the evaporation rate is below 6 ml per day, the treatment may not be successful [[3]].
In North America, lately-deceased beekeeper Bill Ruzicka developed the slow-release MiteGone pads [[4]]. At the normal broodnest relative humidity of 45%-65%, the evaporation rate of 65% formic from a MiteGone pad hit a target minimal release rate of around 6 grams, but at 85% humidity, the evaporation rate dropped to 3 grams a day, and additional pads needed to be added [[5]].
Practical application: Note that for the products above, the recommended daily rate of release of 65% formic was in the 10-20 g range, as compared to the recommended 60-80 g (or more) for an overnight flash treatment.
Years ago, Medhat Nasr and David VanderDussen developed a formic-saturated pad in a plastic bag punched with ventilation holes. NOD Apiary Products later marketed them as Mite-Away, and later Mite-Away II pads, designed to be applied in a spacer rim above the cluster. NOD later replaced that product with slow-release strips to be placed directly within the cluster (to avoid the need for a rim), first releasing MiteAway Quick Strips, and more recently Formic Pro (which has a longer shelf life).
Over the years, I’ve tested the above products, and collected data on their rates of release of the formic acid vapors (Figure 1).
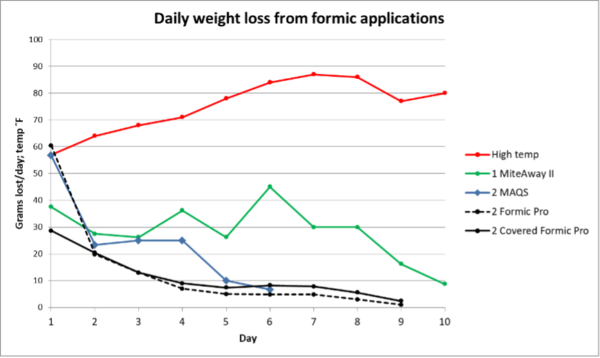
Fig. 1 Mixed data on mean daily weight loss from various NOD Apiary products. High-temperature, MAII, and MAQS data are from 2016; Formic Pro data from 2021 (at highs of ~95°F). Note how the early MAII pads, designed to be applied in a rim above the cluster, gave a fairly steady daily release of 30-40 grams, compared to the later strips, which give a more-intense formic “flash” the first day, then a rapidly-dropping rate of release on the ensuing days.
Practical application: The initial flash of vapors from MAQS or Formic Pro are associated with queen turnover or loss when applied at above the recommended maximum temperature for application (85°F) (From the label: ”Hot temperatures (>92°F) during the first three days may cause excessive brood mortality and queen loss”). But note that by covering the Formic Pro strips with their wrapper, that first-day flash can be eliminated (solid black plot). Our experiments last month suggested that partial covering of the strips might be a way to avoid queen issues, while still obtaining reasonable efficacy against varroa.
Add: The old MiteAway II pads gave a more consistent and extended offgassing of formic acid. I’m not sure that the new products are actually an improvement!
Since we are stuck with increasingly hot summers, we may need to get creative to be able to utilize formic acid during summer weather. I’m unable to test under conditions of high humidity, but followed up on the exploratory research detailed in my previous article — specifically, what would happen if we further reduced and extended the release of the vapors?
Our Follow-Up Experiments
My helpers and I, encouraged by the minimal amount of queen turnover when we covered single Formic Pro strips with their wrapper, wondered about applying two strips at once, but greatly restricting their evaporation rate by leaving them in their original wrapper with only the ends cut off. This would greatly reduce the amount of evaporative surface area, and perhaps provide a low-intensity extended release similar to the Nassenheider or MiteGone delivery methods.
We tried two different application methods (Figures 2 & 3), comparing them to the covered strip method detailed last month.
Note and Objectives: These experiments were not according to label directions. This was exploratory research to (1) observe colony response to a slow-release application of formic acid, and (2) determine whether it was able to substantially reduce the mite infestation rate. We had a limited number of high-mite colonies to work with, so were not able to run tests on as many hives as we would have liked.

Fig. 2 In three colonies, we left the two strips within their original wrapper, with only the two ends cut off, placing them directly over the brood combs in the lower brood chamber.
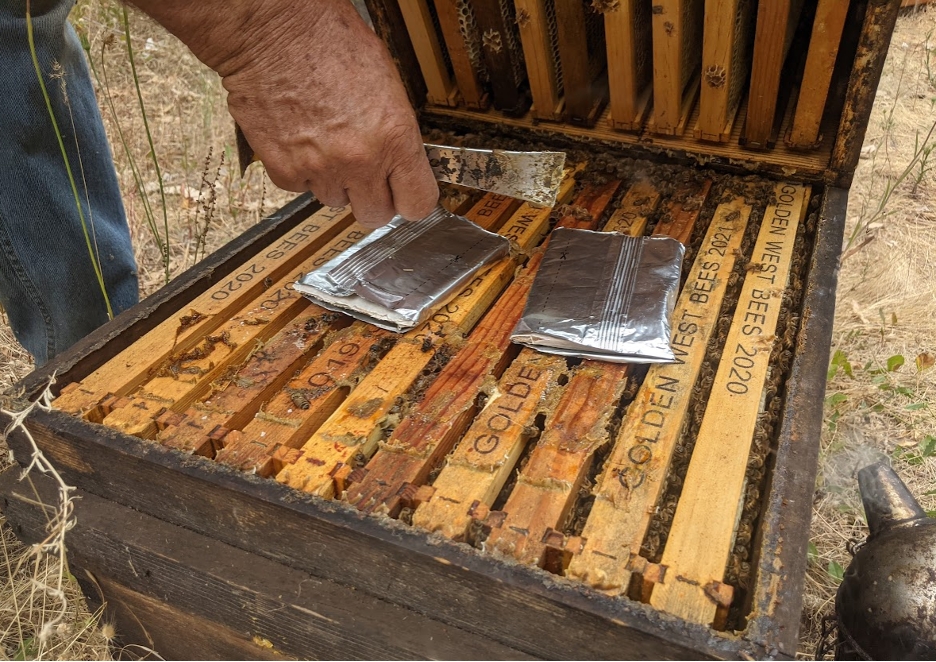
Fig. 3 After observing minimal colony disruption due to the previous treatment, the next day we doubled the amount of evaporation area in seven additional colonies, by cutting the packages of strips crosswise, which also better spanned the brood area.
As positive controls, we also treated five colonies with a single unwrapped strip (outer membrane intact), loosely covered on top with its wrapper, and treated a single colony with two separated unwrapped Formic Pro strips, also covered with wrappers.
For all the colonies, we restricted the entrances to see how they would handle the various application methods in hot weather (Figure 4). I inspected the hives daily for the first several days after application (Figures 5 & 6). The experiments were performed in late July through mid-August, with the daily temperature highs in the mid-90s. The colonies were in double or triple deeps, and varied in strength. There was a moderate nectar flow on, which may have raised cluster humidity. We performed follow-up mite washes after 21 days.
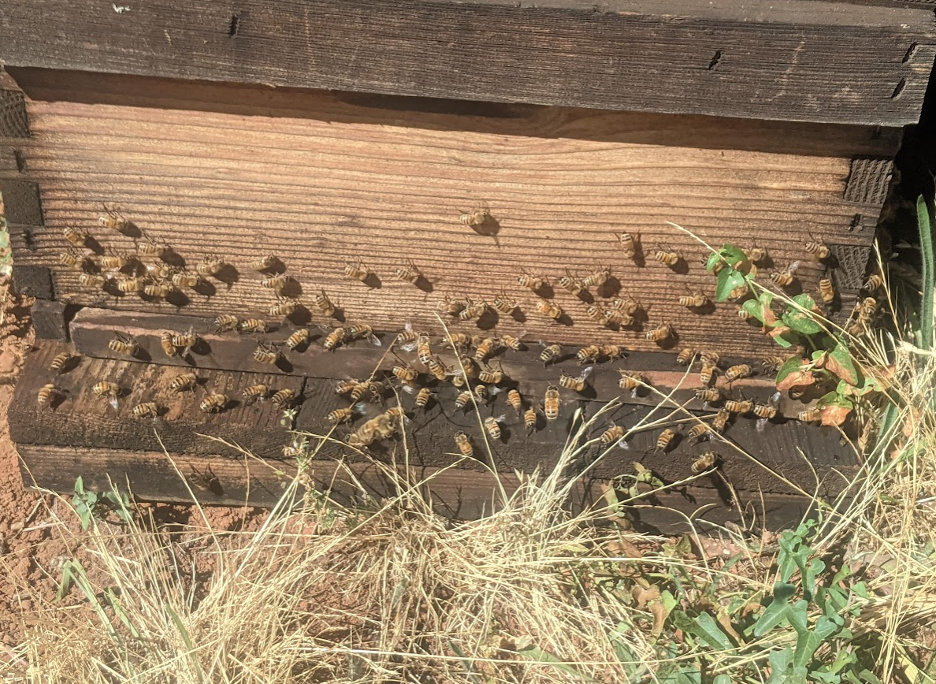
Fig. 4 We inserted wedge-type entrance reducers with a single 3/8” x 3” opening. For the unwrapped but covered strips, there was noticeable bearding the first couple of days. But for the wrapped strips, the photo above was typical of the maximum amount of bearding on the first day, with no noticeable bearding or fanning afterwards.
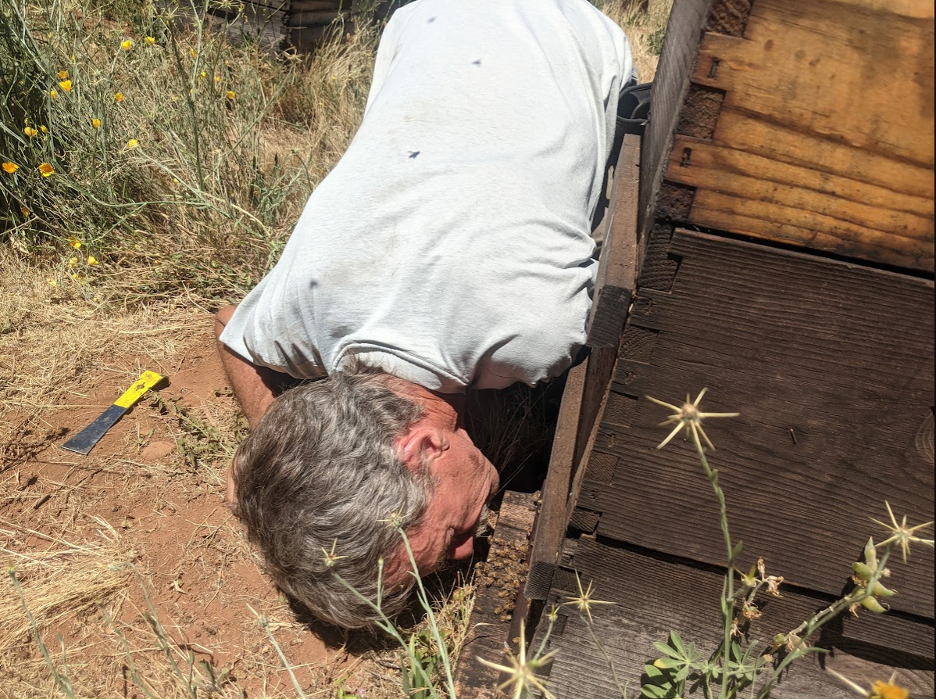
Fig. 5 Formic vapors often kill any vegetation immediately in front of the entrance of a treated hive, but during our experimentation, the grass was already dried up. For the first several days, I checked for the odor of formic vapors in the exhaust from the reduced entrances — formic fumes were strong only from the colony treated with two covered strips, and noticeable for the single covered strips. I was surprised that there was no obvious formic odor exhausting from the entrances of any of hives containing still-wrapped strips, even on the day after application.
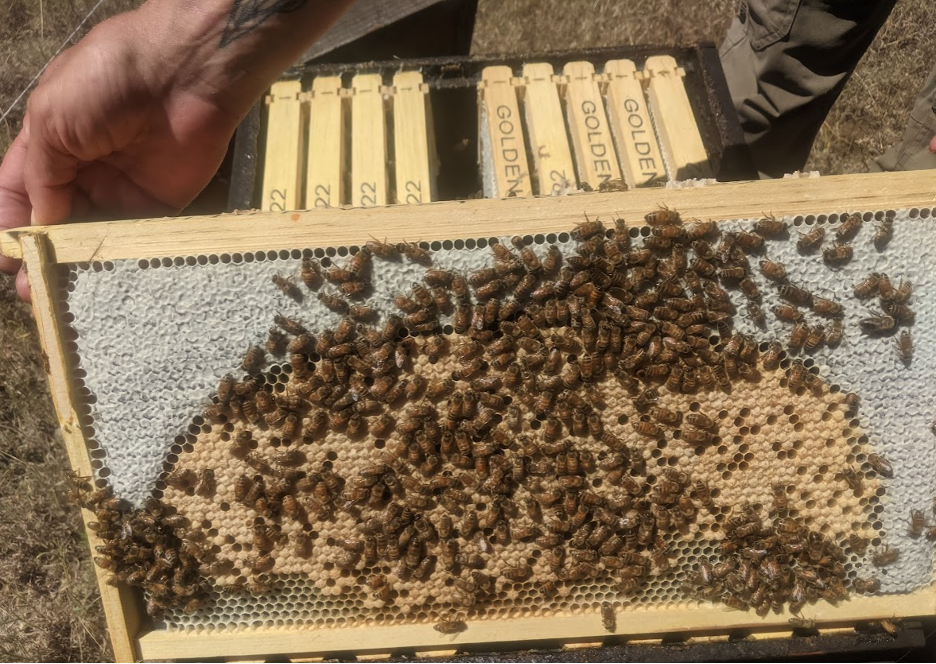
Fig. 6 The colonies with the wrapped strips initially reduced broodrearing a bit, but continued to store honey, and quickly reestablished healthy broodnests.
Of interest, when we removed the wrapped strips after 21 days, was that they were still quite moist within the wrappers, and still smelled of formic acid, but not very strongly. It appeared that the acid had evaporated more than had the water. Due to the remaining water in the strips, I was unable to calculate the amount of formic acid released during the 21-day treatment intervals.
But Did the Wrapped Strips Kill Mites?
OK, the colonies tolerated the wrapped strips just fine. Unfortunately, the slow-release treatment didn’t appear to bother the mites very much either. I’ve plotted the results in Figure 7.

Fig. 7 Treatment of one colony with two strips, separated and covered with their wrapper material, was quite efficacious (resulting in an ending mite count of zero). A single application of only one covered strip to five colonies, resulted in a “knockback” of the mite infestation rates in the ballpark of 45% (indicating that a second application would be required to obtain full efficacy). But there’s too much red showing for either of the slow-release treatments involving strips still wrapped in their packages, with an overall mite reduction for the ten colonies of only 12%.
Not unexpectedly, only one colony in the wrapped strips groups was queenless at 21 days. Perhaps of interest is that the 2-strips covered colony, although still queenright, had supersedure cells (not unusual for a 2nd-year queen).
Discussion
Formic Pro works fine when applied according to its label instructions at recommended temperatures — these experiments were to see whether we could reduce queen turnover when formic treatment is applied in hot weather, while still attaining desired mite reduction by slowing the rate of vapor release.
There were too few colonies involved in these quick and dirty experiments to draw firm conclusions, but the rate of formic release from Formic Pro strip packages with only the ends cut off appeared to be insufficient for varroa control. However, partial covering of Formic Pro strips to reduce the first-day flash off, may in hot weather may be a means of minimizing queen turnover or loss. If you try this yourself, I’d be interested in hearing your results!
Citations and Notes
[1] Amrine Jr, J, & R Noel (2006). Formic acid fumigator for controlling varroa mites in honey bee hives. International Journal of Acarology 32(2): 115-124.
[2] Messin’ With Varroa 2014 – Scientific Beekeeping
[3] The Nassenheider Evaporator (dave-cushman.net)
[4] MiteGone for effective control of the varroa & tracheal mites
[5] Treatment Modification March 21 Y doc (mitegone.com)
Contents
Formic for mite management 1
A trick for using formic pro on weak colonies 2
Queen loss in hot weather 5
Why would queens be more susceptible to formic than workers?. 6
A test of queen loss in tiny colonies. 7
Taking advantage of opportunity. 10
Trial #1 — Would foil covers reduce queen loss or turnover?. 11
Trial #2 — Does covering the strips affect efficacy?. 12
Results. 13
Trial #1. 13
Trial #2. 14
Wrap up. 15
Citations and notes 15
Formic Pro and Queens in Hot Weather
First published in ABJ in September 2022
Randy Oliver
ScientificBeekeeping.com
Formic acid has a lot going for it when used for varroa management. A recurring question is how to avoid the common complaint about queen loss, especially when applied during hot weather. I recently had the chance to run three impromptu “quick and dirty” experiments to investigate.
Formic for mite management
Beekeepers worldwide have long used formic acid — applied by various methods — for control of varroa. Formic is able to achieve rapid mite reduction — and notably, kill mites beneath the cappings — while not contaminating the combs or honey. My sons and I use it extensively in our operation (in rotation with oxalic acid and thymol), and have experimented with a number of application methods, including Formic Pro strips.
Since the recommended temperature range for application of Formic Pro lies between 50 and 85°F (10-30°C), using it during summer may be problematic. There is a common concern about formic causing queen loss (or inducing queen turnover) during hot weather.
Practical notes:
- In my 2020 hot-weather trial [[1]], although a number of colonies replaced their second-year queens after treatment with Formic Pro, 28 out of 29 had a laying queen a month after the second application of a strip.
- Formic acid is great for springtime treatment, since its induced brood break may help to avert swarming, and colonies can easily replace a lost queen at that time of year.
- The release rate of formic acid from Formic Pro is essentially the same as that from Mite Away Quick Strips [[2]], so the results of the experiments below would likely apply to either type of strip.
A trick for using formic pro on weak colonies
I routinely treat nucleus colonies with Formic Pro strips, even in hot weather. A trick for preventing queen loss is to press the hive cover down tight onto the top of a half strip (Figure 1).
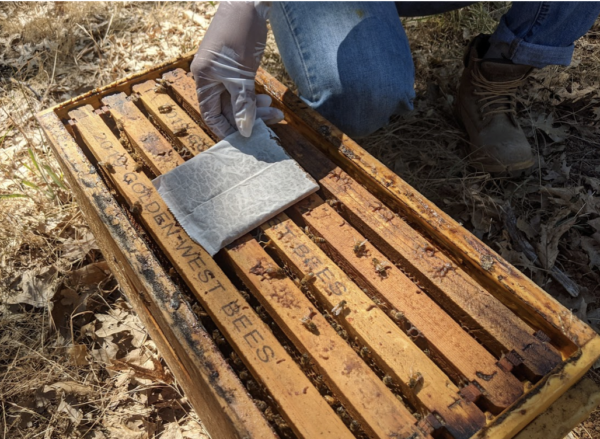
Fig. 1 A nuc can be treated with a half strip of Formic Pro, if the top surface is tightly pressed on by the hive cover.
Take a look at the photo above. Note that evaporation from the bottom side of the strip is largely blocked by the top bars. If evaporation on the upper surface is blocked by the hive cover, that leaves only the outer edges and two 3/8” strips from which the fumes can escape. This greatly slows and extends the release rate of the formic acid. Unlike strips placed between two brood chambers, which rapidly gas off and dry to stiffness, strips placed directly under the cover as above, will even after 10 days of hot weather still be soft and outgassing formic acid (Figure 2). It’s important to press the hive cover down firmly over the strip (Figure 3).

Fig 2 Tammy giving Rose her first introduction to formic vapors. These strips had been in hives for 10 days, with their foil cover placed on top. As you can see from the grimace on Rose’s face, there were still fumes being emitted. The ladies are standing at our mobile mite wash station.
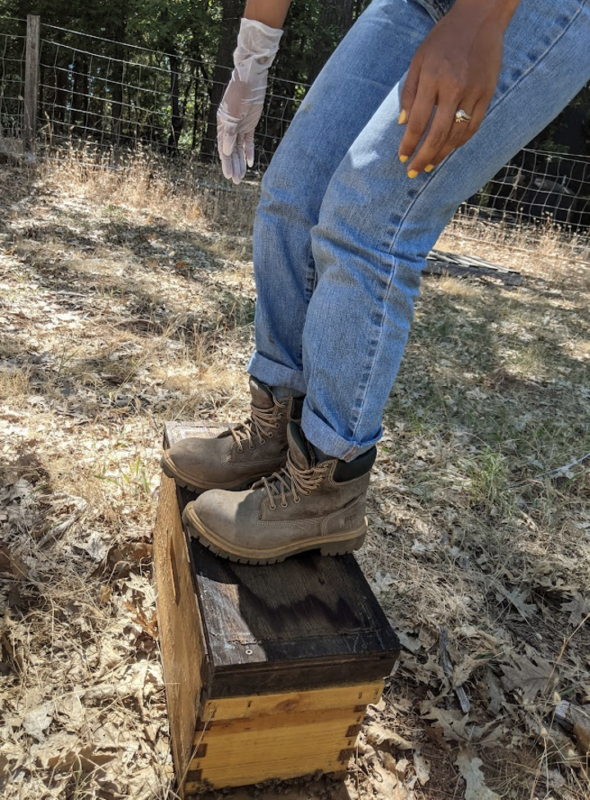
Fig. 3 Helper Rose Pasetes performing a balancing act while demonstrating the pressing method that we use for the nuc cover. Caution: this dangerous technique should only be used with appropriate safety equipment to prevent falls [[3]].
Practical application: The label directions for treatments don’t necessarily cover all the “tweaks” necessary for optimal application under various conditions and situations. Small adjustments to an application method can make a big difference!
Queen loss in hot weather
Despite the temperature recommendations, during our increasingly-hot summers, we still sometimes need to get the mite count down quickly in a colony, and formic fits the bill. We often apply a single Formic Pro strip during hot weather for a “knockback” treatment while honey is still on the hive, and don’t observe queen problems. And once we’ve pulled honey, we routinely break high-mite colonies with second-year queens down into singles, and then blast them hard with a strong formic treatment when the temperature is in the high 90s. A handful of (presumably weak, old, or newly-emerged) dead workers in front of the hive the next day indicates that an adequate dose was applied, but surprisingly, even with these extreme formic blasts, the (unwanted) queens still often survive!
Why would queens be more susceptible to formic than workers?
So why, of all the bees in the hive, would the well-fed and long-lived queen be the most susceptible individual to formic acid? It’s easy to observe that queens simply move away from irritating fumes. I’ve long been curious about an observation by Amrine & Noel [[4]], during their development of the 50% formic “flash” treatment:
Essential oil components in Honey-B-Healthy [consisting of spearmint and lemongrass oils] modify the effect of Formic Acid (FA) treatment on bee hives, such that queens are not lost. This aspect is extremely important for any beekeepers using formic acid to treat varroa mites.
The idea for using lemongrass oil may have come from a prior study by one of Amrine’s students. Vargas-Sarmiento [[5]] found that although application of lemongrass oil could cause queen supersedure on its own, it appeared to suppress the removal of foreign material from the hive.
To my great surprise, I can’t find follow up research on using essential oils to mitigate queen loss when using formic. This is especially surprising because both oils exhibit miticidal properties in their own right [[6],[7],[8]], so adding them might also improve the efficacy of formic acid treatment.
Practical application: Could it be that the queen loss associated with formic treatment is due to the workers, in response to the stress from formic vapors, killing the queen themselves? Or perhaps, since formic fumes kill all the eggs and very young larvae, is the resulting sudden reduction in young brood pheromone a cue that triggers the bees to supersede an apparently failing queen?
And could lemongrass oil, which contains some of the same components as orientation pheromone, suppress such behavior? I’ve been wanting to test the effect of adding these essential oils to formic treatment for some time, but first need to determine an application method that consistently kills queens.
To that end I’ve placed queens into push-in cages directly below formic applications, and found that even if they were constrained from moving away from the fumes, some queens survived even when workers in the same cage died (Figure 4).
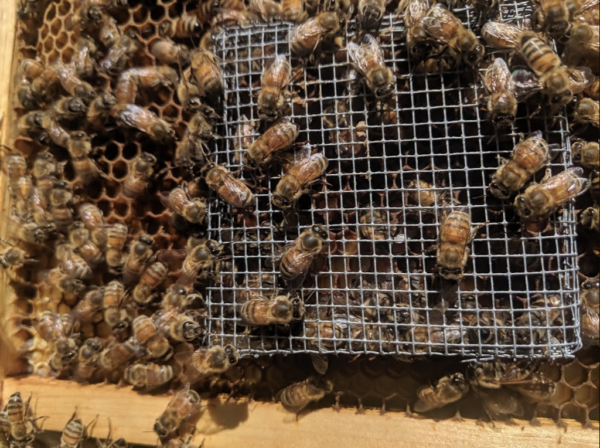
Fig. 4 You can see the dead workers in this cage, but I was unable to get the still-living queen to pose for the photo. It surprised me to find that queens in push-in cages could sometimes survive being placed directly below strong formic applications, with or without attendants in the cage.
And then in June I had an unplanned opportunity to run a spontaneous test…
A test of queen loss in tiny colonies
Assisted by Tammy Hayden and Rose, I was setting up colonies in an outyard for an experiment, when my son Eric called to say that he was heading over to formic blast some “dinks” in the same yard. The colonies (made from splits with queens in their second year) had never built up, and he hoped to both kill the mites as well as the poor queens with the treatment, before adding the boxes to other hives . Realizing an opportunity, I asked him to grab some push-in cages to bring along, but he couldn’t find them, so brought some JZs BZs introduction cages instead.
The colonies were all weak and in single deeps. We quickly found and caged the queens, pressing them into a center comb to be replaced directly below a single Formic Pro strip (Figure 5).
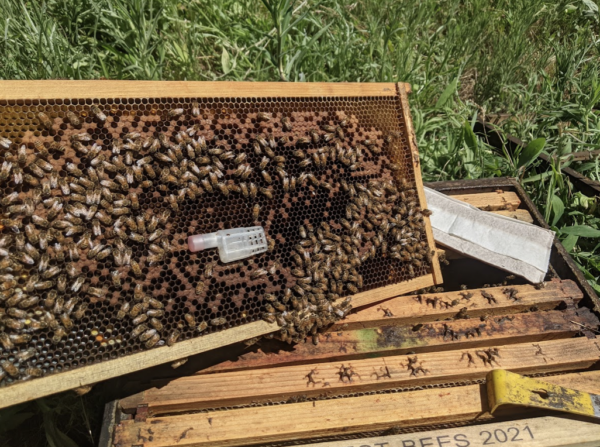
Fig. 5 These “dink” colonies all had second-year queens, and most had poor brood patterns. The weather was hot and dry. We placed the queens in cages without candy or attendants (which if we’d had the push-in cages, we’d have done differently).
Practical application: Many of us have noticed that formic tends to “knock out” poor queens more so than young high-quality queens. So we’re not concerned about the loss of poorly-performing queens.
Not wanting to kill the brood in the weaker colonies, and knowing how the pressing of the lid on top of a strip reduces queen loss, the boys placed 1.5” rims only on the stronger colonies (Figure 6) (to allow for more evaporation from the strips. This proved to make a big difference.

Fig. 6 Rose placing a strip in a 1.5” spacer rim. For the weaker colonies, we pressed the migratory cover directly on top of the strips.
Important note: All hives used in the trials in this article had unrestricted 3/4” high entrances.
We checked two days later for queen survival (Table 1).

Table 1. Note the higher survival rate of the queens in the weaker colonies. I can’t say for sure, but the variable of restricting evaporation from the top of the strips appeared to make a difference.
Practical application: Had Eric been able to find push-in cages, I would have supplied the queens with food and attendants. So I can’t say whether the queens died from the formic fumes, or due to not being fed through the cage openings, but there was clearly a direct or indirect effect of formic upon some queens. I still need to test for the effect of adding essential oils.
The above was not the only opportunity to arise for an unplanned experiment…
Taking advantage of opportunity
Shortly after the above experiment, my helpers and I had taken final mite wash counts from colonies in two different trials (one yard having been treated with different kinds of oxalic dribbles, the other with an experimental thymol gel). Since some of the mite counts were still high at the end of the experiments, we planned to hit those colonies immediately with formic acid.
It occurred to me that those ending mite counts could serve as starting counts for new field experiments with formic, if we started them immediately. So I made the spur of the moment decision to take advantage of this opportunity to follow up on my observation of the substantial amount of queen replacement that occurred in my testing of Formic Pro in hot weather last year.
Practical application: Bees initially move away from formic fumes and fan, but very quickly get used to the odor (and presumed irritation), and even walk right over active strips. Queen “turnover” appears to be due to the initial high-intensity “flash” of vapors that occurs with most application methods. Perhaps by controlling the initial “flash” (similar as to how we treat nucs) we may be able to reduce queen issues.
Experimenting with ways to reduce the initial flash, I thought of using the foil wrapper that Formic Pro strips come packaged in to simply cover the upper surface of the strip when applied between two brood chambers. So last year I measured the rate of weight loss of a couple of pairs of Formic Pro strips placed in a double deep of drawn combs (without bees, to avoid both the variable of fanning, as well as weight gain from propolis deposition) during hot weather, covering one of each pair with its foil wrapper. The results are shown in Figure 7.
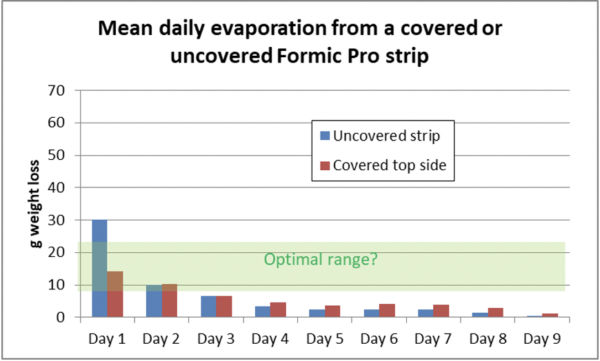
Fig. 7 Covering the upper surface of a strip with its wrapper reduced its daily weight loss (from the release of the formic vapors). This greatly reduced the first-day “flash,” and extended the lower-level release afterwards. Could this method of application improve the treatment?
Practical application: Over the years I’ve measured the daily release rate of formic acid from various application devices and methods. After extensively reviewing published and unpublished studies, it appears that the optimal continuous-release application rate for 65% formic acid is somewhere in the range of 8-22 g per day (as indicated in Figure 6), depending upon ambient temperature, colony strength, and entrance restriction. That said, we’ve treated a lot of hives with the old Mite Away II strips, which released 30-50 g per day, and overnight flash treatments of up to 90 g of 50% formic [[9]].
Back to us having two yards with starting mite counts, it occurred to me that this was an opportunity to test covering the strips in colonies with bees to see whether it reduced the amount of queen turnover. So we started two ad hoc, hastily-planned experiments — one focusing upon queen loss, the other focusing upon efficacy.
Trial #1 — Would foil covers reduce queen loss or turnover?
We ran both trials starting on June 24, during our honey flow. All colonies had been started from nucs with second-year queens. Due to awful weather during their buildup period, the colonies were still relatively weak, all in double deeps with the brood in the lower box, and the bees just starting to work into an upper box containing frames of foundation (we gave a few of the stronger colonies a honey super at the second formic application). Helped additionally by Corrine Jones, we applied formic strips, centered over the clusters, so the combs with brood got direct exposure to the fumes (Figure 8).
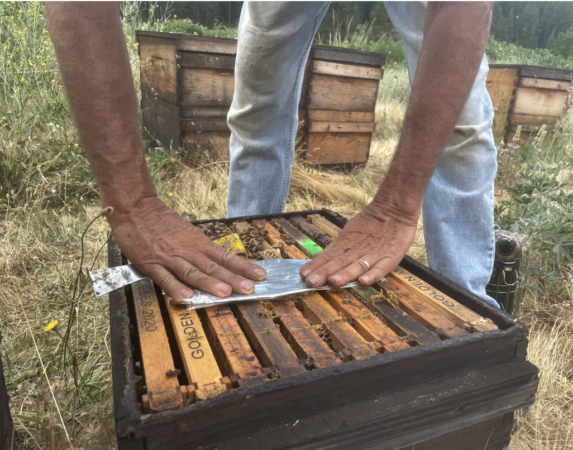
Fig. 8 We ran this trial during hot weather, with daily highs often in the upper 90s (35C+). The colonies in this yard were the stronger of the two, and had higher mite counts, so we wanted to give them a double application of Formic Pro, with a second strip applied after 10 days (replicating the 2020 field trial). In this yard, we covered the tops of the Formic Pro strips with their wrapper in all the colonies in order to reduce evaporation from the upper surface (I opened the wrappers carefully and cut each in half).
Trial #2 — Does covering the strips affect efficacy?
I hadn’t planned either of these spontaneous trials, and made up the protocols as we were driving to the yards. The colonies in the second yard had lower mite counts overall, so in this yard we ran Test and Control groups — with the strips either covered or uncovered. I haphazardly assigned treatments as we applied them, using the full wrapper, pressed flat, to cover one of each pair of strips. Once home, I noticed that based upon the May counts that were still on the hives from the previous experiment, that I’d wound up over-favoring the lower-mite colonies with the uncovered strips (so they could not be used as proper Controls in order to calculate efficacy).
Sampling timing explanation: Since what I was interested in with this experiment was the effect of covering the strips upon efficacy at mite reduction, we only treated once, taking final mite counts 21 days after application of a single strip, which allowed for mites that survived treatment while they were in the brood to emerge, and reestablish the equilibrium of the proportion of mites in the brood relative to those on adult bees, so that a mite wash of adult bees would again reflect the overall mite population in a hive. Details, details, details…
Results
Trial #1
Unlike what occurred in my hot-weather trial of Formic Pro in 2020 [[10]], in which there was a goodly amount of queen “turnover,” in this experiment, every single queen was still alive and had plenty of young brood 10 days after the application of the second strip. Go figure!
As far as reduction in the mite infestation rate of the adult bees, the mean (as well as the median) reductions in mite counts were around 54%. This figure is roughly the same as we obtained in 2020 (85% median reduction, 54% mean). Since the colonies varied considerably in strength, I sorted the results by ending colony strength (Figure 9), to see whether cluster size affected mite reduction.

Fig. 9 I didn’t run a control group, so can’t compute efficacy, but can show the degree of mite count reduction at 21 days (10 days after application of the second strip), by which time most remaining live mites in the brood would have emerged. On average, mite counts dropped by slightly more than half, and were reduced in nearly all the colonies, but there was considerable variation. Note that you see less red relative to blue on the left, indicating that the treatment tended to be somewhat more efficacious in the weaker colonies, in which most of the cluster was located below the strip.
Practical applications: Once again there is great hive-to-hive variation in the amount of mite reduction due to this treatment method. But unlike as in 2020, not a single queen got replaced. But was that due to covering the strips?
Trial #2
In this trial, with only a single strip applied to relatively weak colonies, at 21 days after application, we lost no queens in the 11 covered-strip colonies. But surprisingly, we lost only 1 queen out of 12 in the uncovered strip group (in one of the stronger colonies). So it’s hard to attribute the lack of queen loss in Trial #1 to covering the strips.
Practical application: I have no idea as to why we didn’t see as much queen turnover this year, but covering the strips didn’t appear to make much difference in relatively weak colonies with plenty of room in the second brood chamber.
So how about the effect of covering the strips upon efficacy in mite reduction? Three weeks after applying a single strip, both groups in this yard averaged roughly the same mite counts that they started with, but again with wild variation (Figure 10).

Fig. 10 As far as mite reduction, we didn’t get much from a one-strip “knockback” treatment. There was again considerable hive-to-hive variation, and again I have no idea as to why. I hesitate to draw any conclusions as far as efficacy against varroa, since these were relatively weak (and well-ventilated) colonies.
Wrap up
Allow me to first make abundantly clear that in the above experiments we were applying Formic Pro at well above the recommended temperatures on the label, so its relatively moderate efficacy would not necessarily reflect its performance when applied at lower temperatures.
That said, we observed only a single queen being lost out of 47 treated colonies. I have no explanation as to why our colonies, treated with the same application of Formic Pro, in similar yards at similar temperatures, had such different rates of queen turnover when replicated in two different years.
Nor do I understand the inconsistency in mite reduction with Formic Pro, but suspect that it has something to do with colony ventilation. Once ambient temperature exceeds broodnest temperature (95°F; 35°C), colonies are heat stressed and must increase fanning and evaporation within the hive (but varroa mites are also stressed above this temperature [[11]]). Thus the hive-to-hive variation in mite reduction may have to do with differences in fanning behavior.
There appears to be a fine line, especially during hot weather, between the intensity of exposure to formic acid that it takes to kill the mites under the cappings, and that which causes queen issues. Underwood’s [[12]] research suggested that “queens are affected by acute rather than chronic exposure to formic acid,” and this observation has been confirmed by many others. The trick for formic application appears to be to slow down the initial flash of vapors, while providing an extended lower level of fumigation to chip away at the mites. Along this line, we did so by covering the strips with their wrappers. As I type these words, I’m thinking that perhaps we should also have reduced the entrances (and may return to those yards today to run another experiment).
Since Formic Pro and Mite Away Quick Strips are the only registered formic acid products in the U.S., and since our summers don’t appear to be getting any cooler, it’s likely worthwhile for us to keep experimenting to figure out how best to use them if we need to attain quick knockdown of mites during hot weather.
Practical note: We’re still experimenting with extended-release oxalic acid. This application method takes about two months to attain full efficacy, and in our informal tests, appears to work well in combination with a simultaneous application of formic acid. We need to collect more data on this…
Citations and notes
[1] https://scientificbeekeeping.com/mite-control-while-honey-is-on-the-hive-part-3/
[2] https://scientificbeekeeping.com/mite-control-while-honey-is-on-the-hive-part-3/
[3] Hey, I gotta cover my butt against lawsuits.
[4] Amrine Jr, J, & R Noel (2006). Formic acid fumigator for controlling varroa mites in honey bee hives. International Journal of Acarology 32(2): 115-124.
[5] Vargas-Sarmiento, M (2000). Essential oil treatments to control Varroa destructor Anderson and Trueman 2000 (formerly Varroa jacobsoni Oudemans 1904)(Mesostigmata: Varroidae). West Virginia University.
[6] Islam, N, et al (2016) Management of Varroa destructor by essential oils and formic acid in Apis Mellifera Linn. colonies. Journal of Entomology and Zoology Studies 4(6): 97-104.
[7] Ariana, A, et al (2002). Laboratory evaluation of some plant essences to control Varroa destructor (Acari: Varroidae). Experimental & Applied Acarology 27(4): 319-327.
[8] Refaei, G (2018). Comparing effect of plant-derived oils on Varroa destructor Infesting honeybee, Apis mellifera. Acarines: Journal of the Egyptian Society of Acarology 12(1): 61-64.
[9] https://scientificbeekeeping.com/messin-with-varroa-2014/
[10] https://scientificbeekeeping.com/mite-control-while-honey-is-on-the-hive-part-2/
[11] https://scientificbeekeeping.com/a-test-of-thermal-treatment-for-varroa-part-1/
[12] Underwood, R & R CURRIE (2007) Effects of release pattern and room ventilation on survival of varroa mites and queens during indoor winter fumigation of honey bee colonies with formic acid. Canadian Entomologist 139: 881–893.
Selective Breeding for Mite Resistance, Part 3
Shifting the Genetics of a Breeding Population
Randy Oliver
ScientificBeekeeping.com
The alleles necessary for varroa resistance already existed in my stock of bees, so I didn’t need to “create” anything new. What I’m attempting to do via strong selective pressure is to (1) eliminate from our breeding stock the alleles that favor varroa, (2) increase the prevalence of allelic combinations that confer mite resistance, and (3) “fix” those alleles in the genome of our breeding population. During this process, I want to (4) minimize any deleterious “bottlenecking” of our stock’s genetics. So let’s talk about those genetics.
Several important terms
Breeding population: a population within which free interbreeding takes place and evolutionary change may appear and be preserved.
Genome: The entire genetic structure of an individual or breeding population. All the individuals have the same genes, but the breeding population may contain a number of different forms (alleles) of any gene. In this case, we’re selecting for combinations of alleles that work together to confer mite resistance.
Genotype vs phenotype: An organism’s genotype is the set of alleles that it carries. An organism’s phenotype is all of its observable characteristics — which are influenced both by its genotype and by the environment. We’re selectively breeding for the phenotype of exhibiting very low mite counts; we can only guess at the genetics involved.
Fixation: In population genetics, fixation is the change in a breeding population so that only one allele of a specific gene remains in that population (such as the recessive allele that results in the cordovan phenotype).
Genetic bottlenecking: A reduction of allelic diversity in a breeding population. In the case of our selective breeding, we are intentionally trying to capture and bottleneck the diversity of the alleles associated with the trait of mite resistance (favoring the alleles that confer resistance), but want to conserve the diversity of the rest of genome.
Recurrent selection: A cyclic selection process that is used to increase the frequency of desirable alleles for a character or trait that already exists within that breeding population at low levels. The method involves repeated selection of, and breeding from, generation after generation of individuals exhibiting the desired trait. The interbreeding of their offspring then allows for novel genetic recombinations to occur, and ideally to eventually become “fixed” in that breeding population, without excessive reduction of overall genetic diversity within that population.
Practical application: We’re using the tried and true method of recurrent selection — breeding only from the queens of colonies that exhibit resistance to varroa — in the hope that we can fix the genetic recombinations that confer mite resistance into the genome of our entire breeding population.
The drone pool and avoidance of excessive inbreeding
Selective breeding of honey bees requires the genetic management of an entire breeding population, in this case, the thousand-plus colonies of our own stock dedicated to our program (since we replace every queen in our operation each year with one from our selected breeders). We bring our colonies back from almond pollination chock-full of drones, and flood our mating yards with the sons of last-season’s queens. We also provide free queen cells of our stock to nearby hobby beekeepers. Based upon research by Hellmich [[1]], I have reason to believe that we control most of the genetics of our drone pool.
Practical application: The 50+ bee yards in our operation are largely in wooded areas (which provide suitable cavities for feral honey bees). And although we largely manage swarming, in some years swarms do fly off and establish feral colonies. I do not know how much genetic difference there is between our local ferals and our managed stock, but the influence of our drones upon feral genetics would likely be considerable.
Any of our swarms that successfully establish, but don’t carry the alleles for strong mite resistance, will become feral “mite factories” in late summer. My own yet-unpublished data suggest that they account for much of the mite immigration coming into our managed hives. The good news is that non-resistant feral colonies will likely perish during the winter, and won’t be sending out drones the next spring, meaning that any drones coming from the feral population will likely carry alleles for resistance.
Another poorly-understood variable observed by Couvillon [[2]], is that drones from some mothers may be disproportionally more successful at actually inseminating a queen. Whether that has any linkage to the alleles for mite resistance is unknown.
Selection vs maintaining Diversity
It’s also important to maintain a large enough diversity of queen mothers each generation. Otherwise, excessive bottlenecking of the genetic diversity of your breeding population will catch up with you, resulting in an inadequate number of sex alleles in the population, and queens laying diploid drones (as well as lack of general genetic diversity to allow for environmental adaptation, and to mask the effects of deleterious recessive alleles). As explained by King [[3]]:
Non-random, imbalanced mating designs in which better parents are crossed more often does increase the per generational gains by nearly 10%. However, there is a cost associated in terms of reduced effective population size.
Practical application: In our breeding program, we graft off a minimum of 30 queens (all having mothered a mite-resistant colony) every year in order to maintain an adequate amount of genetic diversity (remember that every queen carries the genetics of dozens of drones) (Figure 1). I keep an eye on both brood patterns, as well as the wide variation in coloration in our queens as indicators of diversity.

Fig. 1 We maintain any colonies marked as “potential breeders” (due to having very low mite counts) in our outyards along with the rest of our colonies, subject to plenty of mite drift. Only after almond pollination do we move the 30-40 “best of the best” to our home yard (shown above) to provide larvae for grafting. To make “breeder grade,” a mite-resistant colony must come back from almonds chock-full of bees and almond honey. To prevent them from swarming, we reduce them to small singles, and only after grafting add back a second brood chamber back to allow them to regrow.
Trying to figure out the genetics involved
It’s important to understand that the queen is not directly involved in varroa resistance — it is the single or combined responses of the workers of the colony that affect the mites. Keep in mind that a colony consists of as many “patrilines” of full-sisters (also called “supersisters”) as the number of drones that the queen mated with — each patriline potentially exhibiting differences in behavior.
It could be that it takes only a single patriline of daughters to initiate varroa-sensitive uncapping behavior. And that behavior could be due to that patriline of workers inheriting a single dominant allele from their father.
Practical application: I’ve seen over and again that there can indeed be strong, gentle, and productive colonies that are absolutely bulletproof to varroa. But the queen’s genetics may have little to do with the resistance exhibited by the colony, due to the genetic influences of the different drones with which she mated. Thus, unlike the genetic heritability of a trait carried by the mating of a single mother with a single father, a trait exhibited at the colony level may be the result of a large number of different paternal bloodlines of workers all interacting with each other. This means that duplication of the genetics of a resistant colony is nearly impossible with open-mated queens, since there were so many fathers involved — any one of which could have been a rare “wild card.”
Allow me show you a couple of examples…
“Old School” Mendelian genetics
We’ve all heard of Mendelian genetics, and how to figure out the expected results from crosses of the genetics of two parents by using a grid of Punnett squares. Let me give the classic example for pod coloration of pea plants, involving a dominant allele (G) for green color, and a recessive allele (g) for yellow pods (Figure 2).
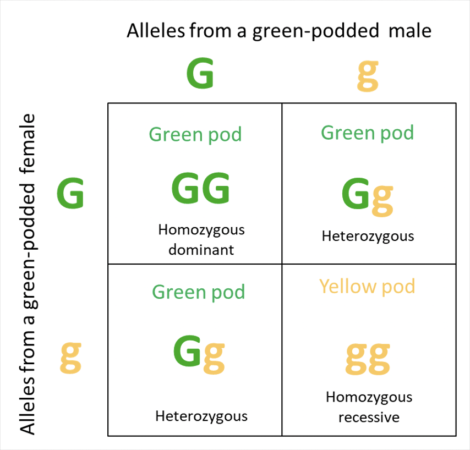
Fig. 2 One of Mendel’s Punnett squares, showing the results a test cross between two pea plants heterozygous for pod coloration, with coloration being determined by the alleles of a single gene. Note that the effect of the dominant allele (G), outweighs that of the recessive allele (g), so that the phenotypes of the parents (the green pods that we see) do not reflect their heterozygous genotypes. Caveat: in light of current knowledge, I’m not sure that pod coloration is actually this simple.
It gets far more complicated with honey bees, because the phenotype of the colony (such as resistance to varroa) may involve the genetics of a large number of parents. A diploid honey bee queen mates with dozens of haploid drones. Each drone thus fathers two patrilines of workers — one carrying the alleles of the drone combined with the alleles of the queen’s father, the other a combination with those of the queen’s mother [[4]]. I’ve illustrated this in the Punnett squares of Figure 3.
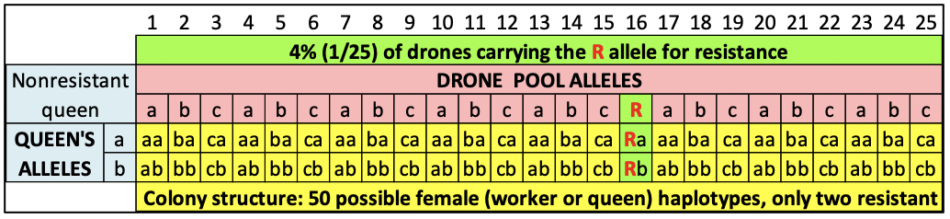
Fig. 3 Let’s hypothetically assume that there is a gene for uncapping behavior with several alleles — a, b, and c being common, but recessive and not triggering uncapping. But there’s also a rare allele R (for resistance) which is dominant, and does induce uncapping behavior. If a non-resistant queen mates with 25 drones, with only one carrying the R allele, this will create a colony consisting of 50 daughter patrilines (the yellow cells), of which only two (the green cells) will exhibit the behavior to uncap cells containing a mite — which may be able to confer resistance to the entire colony as a whole.
Practical application: The colony containing the 50 different genetic combinations of workers shown above might exhibit mite resistance, due to the performance of only the two patrilines of daughters from a single drone. But the queen herself would not carry any alleles for resistance.
If I were to graft daughters from the queen of the resistant colony above, only two out of 50 daughters would carry the R allele for resistance in their maternal bloodline; although some of the rest might be able to exhibit resistance at the colony level by the lucky chance of mating with an unrelated drone that did carry the allele.
Practical application: A great deal of luck is thus involved in picking the right daughters, since we don’t know whether the colony’s alleles for resistance came from the queen, or from one or more drones. It would be easy if the critical alleles were recessive, since the queen of any resistant colony would have needed to have carried that allele, and thus conferred it to half her daughters. But it’s much more difficult to pick a queen actually carrying a dominant allele, since any of the other 48 daughters might head a resistant colony if she happens to mate with a drone carrying that dominant allele.
In the case of selecting for a dominant allele, one must perform “progeny testing” — tracking the performance of the colonies of daughter queens, looking for queen mothers for which half of their daughter colonies exhibit resistance (Figure x), and then going back and breeding off them or the original mother. Last year was the first time that we saw this happen. We’ve got those resistant daughter colonies identified, and plan to move them to isolated mating yards this summer to attempt to “fix” the winning genetics into maternal bloodlines. Keep your fingers crossed!
Figure 4 shows the expected results of what we’d expect to occur if we got lucky (2 chances out of 50) and happened to graft from either of the two daughters above that carried the R allele in their maternal bloodline. In this case, not only would half the workers in her colony carry the R allele (and the colony likely exhibit strong resistance), but half of her daughter colonies would also be expected to exhibit resistance.
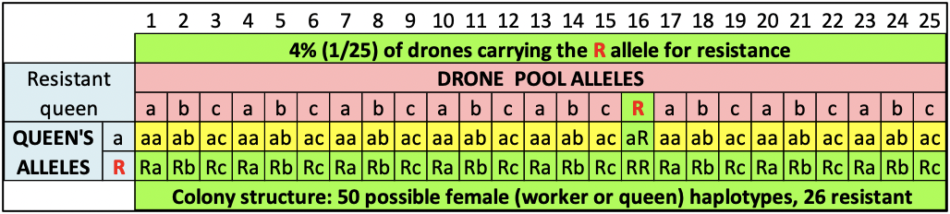
Fig. 4 An example of progeny testing. Same simulation as above, but this time with a queen carrying the R allele for resistance. In this case, fully half of that queen’s daughter colonies would now carry the critical R allele in their maternal bloodline.
Practical application: In one of our yards last season, 24 out of 48 colonies exhibited resistance. We’re crossing our fingers that we may have hit the jackpot!
I’ve spent hundreds of hours these past months running huge Punnett square series (up to 50 x 50 grids) to figure out the genetics involved in order to match our actual progress (shown in my previous article). I’ve run simulations with single alleles, double alleles, dominant or recessive alleles, epistatic dominant or recessive effects, etc. But every simulation suggests that even if the trait came originally from the rare drone, that by breeding only from mothers whose colonies demonstrate full resistance, we “should” be seeing more rapid progress.
Practical application: It’s relatively straightforward to select against a dominant allele with an observable phenotype, by culling individuals expressing that trait (e.g., cull the queens of any “hot” colonies). And it’s pretty straightforward to select for a recessive allele, by breeding only from individuals expressing that trait (e.g., breed only from cordovan-colored queens).
On the other hand, it’s difficult to select for a dominant allele (meaning against other recessive alleles), since you can’t tell whether a queen is carrying a recessive allele unless she’s homozygous for the recessive allele (refer to the pea illustration). See [[5]] for some good visuals of what I’m talking about.
And in the case of the “trait” of varroa resistance, although we might phenotypically observe uncapping behavior, the only proof that it actually conferred resistance to the colony would be to take mite wash counts.
Another reason that the genetics involved are so hard to understand is that the expression of particular traits is far more complicated than simple Mendelian genetics and Punnett square combinations. As explained by Miko [[6]]:
The relationship of genotype to phenotype is rarely as simple as the dominant and recessive patterns described by Mendel… Mendel’s early work with pea plants provided the foundational knowledge for genetics, but Mendel’s simple example of two alleles, one dominant and one recessive, for a given gene is a rarity. In fact, dominance and recessiveness are not actually allelic properties. Rather, they are effects that can only be measured in relation to the effects of other alleles at the same locus. Furthermore, dominance may change according to the level of organization of the phenotype. Variations of dominance highlight the complexity of understanding genetic influences on phenotypes.
Miko goes on to explain the concepts of partial dominance, co-dominance, overdominance, multiple alleles and dominance series. That said, in the simplest cases, one may be able to select for or against some easily-identifiable dominant or recessive traits, such as color, “gentleness,” or hygienic behavior. But mite resistance doesn’t appear to be so simple.
Practical application: The bottom line is that selective breeding for mite resistance is a challenge, especially since there are dozens of fathers involved in any resistant colony. I’ve given up on trying to understand all the complex genetics (and epigenetics) involved in mite resistance. So we just keep plugging away with our “recurrent selection” approach — which has a long history of success. In short, we simply requeen our entire operation each year solely with daughters of queens whose colonies exhibited control of varroa for the entire previous year.
What can small-scale beekeepers Realistically hope to do?
I often get asked by small-scale beekeepers how they could engage in a breeding program of their own. Unless they plan to instrumentally inseminate each of their queens, the question then is to what extent can they realistically expect to control the genetics of their drone pool?
Practical application: Honey bees may be the most difficult animal on Earth to selectively breed, due to the uncontrolled polyandry of the queens with the drones of the surrounding breeding population. The selective breeding experiment that my sons and I are engaged in is targeted for large-scale queen producers who run enough colonies to manage the genetics of an entire breeding population.
As you can see from our results, in which we essentially control the genetics of the drone pool, but also apply extreme selective pressure (which requires a lot of colonies to pick from), that it takes time and dedication.
The reality is that it’s difficult to imagine that any hobby beekeeper (unless they live on an isolated island) could be expected to significantly affect the breeding population surrounding their apiaries.
That said, if they are surrounded by an unmanaged population of native or feral honey bees, upon which Mother Nature herself has impartially applied enough selective pressure (“live and let die”) upon the free-living breeding population to gain resistance to varroa, the beekeeper could work in collaboration with the “natural” evolutionary process (nicely discussed by van Alphen [[7]]).
They could do so by eschewing bringing in non-resistant commercial stock, and instead populating their hives with swarms from the surrounding “wild” population. The beekeeper could then retain only colonies that were amenable for beekeeping (such as being gentle and productive), cull the queens of those that were not, and rear replacement queens from the “good ones.” I get reports from beekeepers in areas with viable unmanaged honey bee populations of doing just that, and being happy with the results.
Yes, such a beekeeper could consider themselves to be a “breeder,” but most of the credit would actually go to Mother Nature, with the beekeeper simply culling out bloodlines from their few managed colonies that were too spicy or swarmy. Nothing wrong with that!
Acknowledgements
I want to express my appreciation to my intellectually-gifted beekeeping friend Richard Cryberg, who has for years been an invaluable resource to answer my deep questions on chemistry, pesticides, biology, disease, and especially animal breeding and genetics. His deep knowledge and experience in the breeding of racing pigeons has helped me immensely in my experiment to selectively breed for varroa-resistant honey bees.
References
[1] Hellmich, R & G Weller (1990) Preparing for Africanized honey bees: Evaluating control in mating apiaries. ABJ 130(8): 537-542.
Hellmich, R, et al (1993) Evaluating mating control of honey bee queens in an Africanized area of Guatemala. ABJ March 207-211.
[2] Couvillon, MJ, et al (2010) Sexual selection in honey bees: colony variation and the importance of size in male mating success. Behavioral Ecology, 21(3): 520-525.
[3] King, J & G Johnson (1993) Monte Carlo simulation models of breeding-population advancement. Silvae Genetica 42(2-3): 68-78.
[4] Recombination and crossover of chromosomes during the process of meiosis complicates this even further — in reality every single worker bee (or potential queen) could theoretically be genetically unique.
[5] Selection for qualitative phenotypes. https://www.fao.org/3/v8720e/V8720E03.htm
[6] Miko, I (2008) Genetic dominance: genotype-phenotype relationships. Nature Education 1(1):140.
[7] Van Alphen, J & B Fernhout (2020) Natural selection, selective breeding, and the evolution of resistance of honeybees (Apis mellifera) against Varroa. Zoological letters 6(1): 1-20.
Contents
The Achilles’ heel of varroa. 2
Genotype vs. Individual- or colony-level phenotype. 2
gene regulation. 3
An example of the differential expression of genes in the honey bee. 4
What Is our own bees’ mechanism(s) for Resistance?. 6
Testing for Uncapping behavior 9
Is there a Cost to the colony for resistance?. 10
Our Traditional Breeding Experiment 11
References. 11
Selective Breeding for Mite Resistance, Part 2
Mite Resistance and Genetic Expression
First published in ABJ in July 2022
Randy Oliver
ScientificBeekeeping.com
A selective breeding program is all about genetics. But humans bred plants and animals for thousands of years before we knew anything about DNA or genetics. What breeders selected for were traits. So how are my own varroa-resistant colonies actually preventing varroa mites from building up in their hives? And why does the trait appear to exhibit low heritability?
I’ve already mentioned that I am selecting for varroa-resistant bees, as opposed to them being merely varroa-tolerant. Resistance to varroa takes place at the colony level. But that colony-level resistance depends upon the interactions of individual bees with individual mites.
It’s easy to visualize individual bees recognizing and attacking mites (so called “grooming” and/or “biting” behaviors), but this agile parasite has evolved to avoid harm. Varroa mites are able to survive for long periods in colonies of their original host (Apis cerana), despite the fact that cerana workers vigorously attack and groom mites not only off their own bodies, but also off their nestmates. So although grooming is certainly a useful behavior to select for, it’s unlikely that it alone would be enough to confer a colony with adequate resistance [[1], [2]].
The Achilles’ heel of varroa
All mites eventually die of old age. For mites to become a problem in the hive, they must reproduce more quickly than they die. Thus, as far as a honey bee colony is concerned, varroa’s Achilles’ heel is its ability to reproduce, especially in worker brood. Thwarting this may require a “team effort” by the bees. For example, a mite-infested larva or pupa might emit an olfactory signal, or even self-sacrifice (“social apoptosis”), a nurse bee would then need to recognize and respond to that signal and initiate uncapping of the cell, and then a different worker might either remove or cannibalize that infested pupa.
My point is that all of the above responsive behaviors are controlled by genetics, and may require the combined efforts of two or more workers (as well as the infested larva or pupa), each carrying different critical alleles (different “flavors” of any gene) that somehow code for the initiation of each specific behavior.
And then it gets even more complicated; it is not enough that a worker carry the right alleles (the worker’s genotype, or “library” of genetic instructions), those genes must then be read and expressed in the worker’s phenotype — its morphology, physiology, and behaviors. Molecular biologists now talk about the “transcriptome,” which reflects the genes that are being actively expressed from the DNA of the genome into different forms of RNA (the transcriptome) at any given time.
Practical application: The RNA in the transcriptome can code for the manufacture of proteins, or have regulatory functions. I suspect that resistance to varroa is gained mainly from shifts in the regulatory aspect of the transcriptome. Some genes being actively expressed in the transcriptome (at the moment) might be triggered by a nurse detecting a specific odor, which may then unleash “regulatory cascades” that result in the initiation of behaviors involved in mite resistance.
The coding and non-coding genes work together. For example, for a nurse bee to detect that a mite is reproducing under the capping of a cell may require an allele that specifically codes for the formation of an olfactory receptor protein which might bind to a specific odor emitted by immature mites (or a cuticular hydrocarbon from an infested pupa). Binding of that specific odor molecule at the tip of the nurse’s sensory papillae could then initiate a behavioral response cascade, resulting in that nurse bee initiating the chewing away of the capping.
Practical application: If you can’t smell it, you can’t respond to it! For a nurse to respond to a particular scent of a mite or an infested pupa, she must possess a specific olfactory receptor protein that binds to that scent molecule. The coding alleles for such critical receptor proteins may be rare in commercial bee stocks. But once detected, the alleles for the behavior of uncapping targeted pupa are already common in any breeding population, so it may take only the slightest genetic tweak to shift non-resistant bees toward resistance.
Genotype vs. Individual- or colony-level phenotype
Mechanisms for varroa resistance take place at both the individual worker (or larva) level, as well as at the colony-as-a-whole level. The phenotype is where the rubber of the genotype hits the road, and is where the end results of any particular assortment of alleles become evident.
Resistance for varroa may involve the individual larva or pupa (by olfactory signals, social apoptosis, behavior, or gene expression [[3]]), or the behavioral actions of individual adults (grooming, uncapping, or hygienic behavior)[[4]], or take place at the colony level (temperature regulation, absconding, or brood management). All the above phenotypes result from the “expression” of the genotype. As summarized by a recent study that investigated SMR and VSH (Suppression of Mite Reproduction and Varroa-Sensitive Hygiene) [[5]]:
All of the above demonstrate that [mite non-reproduction] is a complex mechanism combining multi-factorial effects, such as adult bee behavior, brood and mite physiology, and bee and mite genetics.
Practical application: The above-cited study is open access, and is must reading for anyone engaged in a selective breeding program. The complexity of mite resistance may help to explain why it’s more difficult to breed for than some other traits.
Gene regulation
Left to themselves, honey bees have shown that they can fairly rapidly adapt to varroa. The process of selected breeding is simply human-directed evolution (forcing adaptation by the breeding population to our selective pressure). The fastest and most efficient way for a species to adapt to environmental changes (such as the gaining of a new parasite) is by gene regulation.
The above concept is explained by Boyle [[6]]:
For typical traits, even the most important loci in the genome have small effect sizes and that, together, … only explain a modest fraction of the predicted genetic variance … in contrast to Mendelian diseases — which are largely caused by protein-coding changes — complex traits are mainly driven by noncoding variants that presumably affect gene regulation. [Highlighting mine]
Albert [[7]] found that almost every gene is influenced by a complex set of regulatory regions all over the genome. Some hotspot regions (“expression quantitative trait loci”) were found to influence the expression of thousands of other genes. I suspect that the genetics of breeding for varroa resistance has mostly to do with selecting for the genetic regulation of alleles already existing in our breeding population (since we clearly see some existing colonies that are able to keep varroa in check) [[8]]. As stated eloquently by Alyson Ashe [[9]]:
The possibility of extreme phenotypes must lurk within the normal genome. These extreme phenotypes are only expressed when an environmental or genetic challenge is sufficient to reveal them. Therefore, the selection on the regulation of a gene network alone should be sufficient to produce the same extreme phenotype without change in the average genotype of the genes that directly contribute to a trait.
The Takeaway: The alleles of a few critical genes act as “switches” to influence or initiate regulatory cascades that control the expression of many other genes involved in physiology or behavior. Evolution may not depend upon novel mutations (adding new tools to the toolbox), but rather upon using the existing tools in the genome in new ways. In our selective breeding experiment we’re not hoping for “magic,” but simply selecting for colonies from our own stock that are using their existing toolboxes to effectively control varroa. In other words, simple “old school” selective breeding.
An example of the differential expression of genes in the honey bee
Allow me to offer a graphic example of how the genotype of a honey bee egg can be very differently expressed in the phenotype of the resulting adult. The one-celled zygote formed upon fertilization contains two sets of chromosomes — one from the queen, and one from a drone with which she mated. Let’s focus upon one matching pair of chromosomes in that zygote, which may have received from each parent a slightly different “spelling” (or not) of a single allele at one critical gene. That minuscule difference, coupled with the “environmental effect” of what the larva gets fed, can result in the formation of any of three strikingly different sexual phenotypes during the development of that egg into an adult.
Surprisingly, the default for any fertilized honey bee egg is to become a male (a viable diploid drone) [[10]]. Only if the matching pair of the Complementary Sex Determiner (csd) gene happens to carry two different alleles (out of over a hundred known), will the csd gene then produce messenger RNA to activate the Feminize gene (fem), which will then kick in to initiate a genetic “regulatory cascade” that results in the development of a female bee.
And once differentiated into a female, depending upon the “environmental influence” of how that now-female larva is fed, “she” can develop into either a worker or a queen (Figure x) [[11]]. Also surprising is that the young larval transcriptome is more influenced by diet than by sexual differentiation [[12]].

Fig. 1 A diagram of how a triggered genetic regulatory cascade, or the environmental effect of feeding, can differentiate the expression of the genes in a fertilized egg into developing into a drone, or either caste of female. Credits [[13]].
My point is that any genome has the potential to develop into substantially different morphological or behavioral phenotypes, depending upon which genetic “switches” are flipped. A honey bee embryo exhibiting virtually identical genetics (with only the slightest difference of a single allele at one regulatory gene) has the potential to develop into either a drone, a queen, or a worker. Further regulation of the transcriptome can then shift that worker from acting as a jelly-producing in-hive nurse, to a wax-producing “mid-age” bee, a guard at the entrance, or eventually into a specialized free-flying pollen, nectar, water, or propolis forager.
Practical application: The above is an example of how differential expression of a fixed set of genes can result in vastly different phenotypes (morphologically, physiologically, or behaviorally). Breeding for mite resistance may not be so much about finding novel genes, but rather about selecting for bloodlines that differentially express existing regulatory genes in response to certain cues (such as the odor of varroa mating pheromone, or mite-stressed pupae). Slight differences in how even a single patriline of workers in a colony flip their genetic “regulatory switches” may allow the colony as a whole to exhibit resistance to varroa.
An addition following a Letter to the Editor:
I was in no way trying to suggest that diploid drones were “normal.” Drones are of course normally haploid, coming from unfertilized eggs, and diploid drone larvae are normally quickly consumed by the nurse bees. Since, as you say yourself that “It is fairly common knowledge that the common male drone bee is from a HAPLOID zygote,” I mistakenly assumed that my readers would know that, and it didn’t occur to either me or the Editor that further explanation was necessary.
The point of my example was that any fertilized egg has the “choice” of either of two (as termed by Herrmann1) “sex options” — taking either of two different developmental pathways resulting in the development of either male or female organs and body morphologies. Which pathway it takes is determined solely by whether it carries the same, or a different, pair of alleles at a single gene.
Thank you for bringing this possible confusion to my attention; I will add this correction to the article when I post it to my website.
Regarding your claim that I failed to attribute credit, I make a point of always acknowledging the source of images in my illustrations, as I did for this one in the legend. One cannot cite USDA as an author or illustrator, one must cite the human being who actually created the work, which I did.
1Herrmann, M, et al (2005) Characters that differ between diploid and haploid honey bee (Apis mellifera) drones. Genet. Mol. Res. 4(4): 624-641.
What Is our own bees’ mechanism(s) for Resistance?
I don’t limit the “genetic creativity” of my bees by telling them how to do their job; I just fire all the ones that don’t get the job done. After a few years of strong selective pressure, I observe quite a bit of ”bald brood” (Figure 2).

Fig. 2 “Bald brood,” likely indicating uncapping/recapping behavior, involves some workers (perhaps only a patriline of full sisters fathered by a single drone) chewing the cappings off apparently-healthy sealed pupae, but not necessarily removing those pupae (removal would be termed “Varroa Sensitive Hygiene” (VSH) [[14]]). The pupae may then be capped back over, perhaps by a different patriline of workers (recapping is a common trait).
Uncapping/recapping behavior was described in 1998 in Brazilian Africanized honey bees by Corrêa-Marques and de Jong [[15]], elaborated on by Harris, Danka, and Villa in 2010 [[16]], recently identified as a common trait for resistance in bee populations around the world by Stephen Martin in 2020 [[17]], and elaborated upon by Melissa Oddie in 2021 [[18]].
Since I keep an eye on the first brood patterns of our new queens (in order to look for signs of excessive inbreeding resulting in diploid drones), I note that the first patterns are generally quite solid (Figure 3).
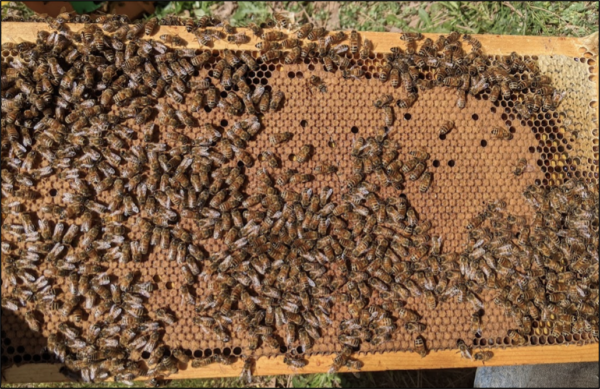
Fig. 3 I monitor the brood patterns of our new queens each year to make sure that we’re not inbreeding excessively, which would result in spotty brood patterns due to diploid drones.
Compare the above to a typical pattern of one of our mite-resistant colonies later in the season (Figure 4).

Fig. 4 Although strong and healthy, with a mite wash count of zero, note the typical presence of open cells in this resistant colony later in the season. I suspect that the open cells have to do with some aspect of mite resistance, such as VSH, perhaps following uncapping behavior.
Practical application: I rarely observed bald brood before varroa, but now see it regularly in our own resistant colonies. But it’s not always easy to observe, because if a colony holds its infestation low, there won’t be many infested cells at any time. But what I do see is that there appears to be some threshold of mite infestation at which uncapping and removal of infested pupae really kicks in, especially in my resistant colonies.
I have noticed that bald brood appears to occur in patches, which is explained by an illuminative paper by Grindrod and Martin [[19]]:
These findings are important as they suggest first that all colonies have the ability to detect and thus potentially to remove mite infested brood, and secondly that whether a cell is checked for Varroa is influenced by the infestation status of its surrounding cells.
Grindrod’s findings suggest that the olfactory cue from mite-infested cells may trigger, at least in certain workers, upregulation of their inspection and uncapping behaviors, similar to how other environmental cues alter bee behavior.
Practical application: It’s not clear whether uncapping behavior or even the removal of infested pupae results in the death of any adult mites in the cells, but it likely decreases their overall reproductive success (varroa’s Achilles’ heel).
Testing for Uncapping behavior
During visual inspection, such as in the above photos, one may see uncapped pupae and/or scattered open cells, but you can’t tell the degree to which cells have been uncapped and then recapped without the removal of the pupae. Finding that out requires removing the cappings over sealed brood, and inspecting for signs of being recapped. When recapped in a dark comb, the underside of the capping of a recapped cell will look darker, due to the light-colored silk cocoon having been chewed away.
This process is tedious to do with forceps. In discussion with other researchers, I’ve tried using molten beeswax and gauze or sticky tape to remove a large number of cappings at once. But neither worked well, for various reasons. Luckily, I got a tip from Dr. Ralph Büchler to try using depiliatory strips [[20]], which work as well to remove cappings as they do for bikini waxing (Figure 5).

Fig. 5 This assay must be performed on capped pupae (capped larvae may not have yet spun their cocoons). Look for a patch of pupae at pink-eyed stage, so that the nurses have had plenty of time to detect any cue for uncapping. It’s easy to pick out the dark recaps, compared to the intact undersides still showing light-colored silk cocoons.
Practical application: I’ve only just begun to experiment with this assay, and may write more about it as I learn more. It could be a quick assessment method for mite resistance. Let me be clear that this is only one mechanism for resistance that happens to stand out in our colonies — I have no idea how many different mechanisms that they are actually using (or whether all bloodlines are using the same mechanisms).
Of course I’m curious as to how our resistant colonies keep varroa under control, but as concluded by Eynard [[21]]:
[Mite Non-Reproduction] measurement remains one of the few measurements for varroa resistance in honey bee populations, which can be achieved in the field on a relatively large scale. Although time consuming and tedious to implement, it also gives a lot of different information which can help us to better understand the control mechanisms that bees use to counteract the varroa mite. [Boldface mine]
In our selective breeding program, we do observe a great deal of uncapping behavior and some VSH. But because a resistant colony contains very few mites, it would indeed be tedious to confirm. I’ll leave it up to someone else to figure out how our zero-count colonies manage to control the mite.
Is there a Cost to the colony for resistance?
One advantage of uncapping/recapping behavior for mite resistance is that there is not as much cost to the colony as from brood sacrifice, such as with “social apoptosis” or Varroa Sensitive Hygiene [[22]]. I don’t see any negative tradeoff in productivity or gentleness in our most-resistant colonies — they’re often the most productive hives in a yard. This could be due to their not having to deal with having their all-important fat bodies getting destroyed by the mites, as well as benefitting from not having mites injecting their weakened bodies with viruses.
Our Traditional Breeding Experiment
It’s clear that mite resistance is attainable, but it may take a while to “fix” the trait in our breeding population [[23]]. We’re gonna stick with Harbo and Harris’s suggestion to simply identify those colonies that by some means are able to prevent varroa from building up over the course of the season [[24]]:
We define mite resistance as the ability of a colony of honey bees to impede the growth of a population of V. jacobsoni. With this definition, a highly resistant colony of bees would cause a mite population to decline and then to either disappear or be maintained at a very low level. This is the breeding objective.
Exactly! Funded researchers can perform the tedious work. I just define the job description for our bees, and breed only from those that do the job, as evidenced by, however they do it, of maintaining mite counts of zero!
References
[1] Vandame, R, et al (2002). Parasitism in the social bee Apis mellifera: quantifying costs and benefits of behavioral resistance to Varroa destructor mites. Apidologie 33(5): 433-445.
[2] Borba R, et al. (2022) Phenomic analysis of the honey bee pathogen-web and its dynamics on colony productivity, health and social immunity behaviors. PLoS ONE 17(1): e0263273. “… our data failed to show any significant relationship between damaged mites and the mite infestation indices measured.”
[3] Conlon, B, et al (2019). A gene for resistance to the Varroa mite (Acari) in honey bee (Apis mellifera ) pupae. Molecular Ecology. 28. 10.1111/mec.15080.
[4] Scannapieco, A, et al (2016). Individual precocity, temporal persistence, and task-specialization of hygienic bees from selected colonies of Apis mellifera. Journal of Apicultural Science 60(1): 63–74.
[5] Eynard, S, et al (2020) Descriptive analysis of the Varroa non-reproduction trait in honey bee colonies and association with other traits related to Varroa resistance. Insects 11(8): 492.
[6] Boyle, E, et al (2017) An expanded view of complex traits: from polygenic to omnigenic. Perspective 169 (7): 1177-1186.
[7] Albert, FW, et al (2018) Genetics of trans-regulatory variation in gene expression. Elife 7:e35471. doi: 10.7554/eLife.35471.
[8] Albert, F & L Kruglyak (2015) The role of regulatory variation in complex traits and disease. Nature Reviews/Genetics 16:197.
[9] Ashe, A, et al (2021) How does epigenetics influence the course of evolution? Phil. Trans. R. Soc. B3762020011120200111.
[10] Gempe, T, et al (2009) Sex determination in honeybees: Two separate mechanisms induce and maintain the female pathway. PLoS Biol 7(10): e1000222. doi:10.1371/journal.pbio.1000222. “in the absence of the female-specifying signal, the male variant is produced that is the default regulatory state.”
[11] The developing worker larva is also affected by the presence of queen pheromone. Woyciechowski, M, et al (2017) Honeybee worker larvae perceive queen pheromones in their food. Apidologie 48: 144–149.
[12] He, X-J, et al (2019) A comparison of honeybee (Apis mellifera) queen, worker and drone larvae by RNA-Seq. Insect Science 26: 499–509. “For young larvae (2-day-old) environmental factors such as larval diet have a greater effect on gene expression profiles than ploidy or sex determination.”
[13] I arbitrarily placed the csd gene on a fanciful chromosome. Bee drawings from: Kauffeld, M (1980) Seasonal cycle of activities in honey bee colonies. In, Beekeeping in the United States, Agriculture Handbook 335: 30-32.
[14] Harris J, R Danka, J Villa (2021) Honey bees (Hymenoptera: Apidae) with the trait of Varroa sensitive hygiene remove brood with all reproductive stages of Varroa mites (Mesostigmata: Varroidae). Ann Entomol Soc Am. 2010; 103(2):146–52.
[15] Corrêa-Marques, M, D de Jong (1998) Uncapping of worker bee brood, a component of the hygienic behavior of Africanized honey bees against the mite Varroa jacobsoni Oudemans. Apidologie 29 (3): 283-289.
[16] J Harris, R Danka, J Villa (2010) Honey bees (Hymenoptera: Apidae) with the trait of varroa sensitive hygiene remove brood with all reproductive stages of varroa mites (Mesostigmata: Varroidae). Annals of the Entomological Society of America 103(2): 146–152.
[17] Martin, S, et al (2020) Varroa destructor reproduction and cell re-capping in mite-resistant Apis mellifera populations. Apidologie 51: 369–381.
[18] Oddie, M, et al. (2021) Reproductive success of the parasitic mite (Varroa destructor) is lower in honeybee colonies that target infested cells with recapping. Sci Rep 11: 9133.
[19] Grindrod, I & S Martin (2021). Spatial distribution of recapping behaviour indicates clustering around Varroa infested cells. Journal of Apicultural Research 60(5); 707-716.
[20] I used Veet brand “Ready-to-use wax strip kit” (a gel adhesive used without heating).
[21] Eynard, S, et al (2020) op cit.
[22] Vandame, R, et al (2002) op cit.
[23] By us continually removing from the breeding population any queens who apparently didn’t carry alleles that conferred resistance to her colony.
[24] Harbo, JR & JW Harris (1999) Selecting honey bees for resistance to Varroa jacobsoni. Apidologie 30: 183-196.
Contents
Resistance vs. Tolerance or “Survival” 1
Managed apiaries vs. natural evolution. 2
Background. 3
The necessity of Mechanical Agitators 3
The resistant colonies 6
So what’s our progress so far?. 10
A built-in lag inherent in open mating programs 13
Exhibit A: Mite-count tracking for our 2022 breeders 14
Coming. 16
References. 16
Walking the Walk
Selective Breeding for Mite Resistance; 2022 Update, Part 1
First published in ABJ June 2022
Randy Oliver
ScientificBeekeeping.com
I’ve been selectively breeding queens for many years. Breeding for color, gentleness, or AFB resistance was easy. Ditto for tracheal mite resistance, as well as for the ability to deal with Nosema ceranae. But breeding for varroa resistance has proven to be more difficult.
The obvious long-term solution to The Varroa Problem is to stock our hives with varroa-resistant bees. Varroa’s original host, Apis cerana, fits that bill. And left to their own, the hard hand of natural selective pressure applied to unmanaged bee populations has resulted in the evolution of resistant bees in various areas. But progress has been slower for those engaged in the selective breeding of managed European honey bees; their worldwide efforts are well reviewed by Le Conte [[i]]. So the question is, why is it taking so long for reliably mite-resistant bees to hit the market?
Resistance vs. Tolerance or “Survival”
Before I get going, please first allow me to clear up some terminology. I often hear people use the terms “resistance” and “tolerance” interchangeably. They actually have two very different biological definitions, as explained by Ayres and Schneider [[ii]]:
A host can evolve two types of defence mechanisms to increase its fitness when challenged with a pathogen — resistance and tolerance … Resistance is defined as the ability to limit pathogen burden while tolerance is defined as the ability to limit the health impact caused by a given pathogen burden. The sum of these two mechanisms defines a host’s defensive capacity.
There are “varroa tolerant” or “survivor” colonies, that may thwart the mite by maintaining tiny colony sizes, or by swarming or absconding frequently, or by fiercely attacking any invaders, but beekeepers are generally not interested in bees with those traits. Or “tolerant” bees may exhibit resistance to the mite-vectored viruses, even at high mite infestation rates.
But what I want are bees that take me back to the (pre-varroa) ”Good Old Days.” So I’m interested in bloodlines that “just say no” to varroa, and are by some means able to prevent the mites from successfully reproducing in their colonies.
Practical application: I’m breeding for resistance, not tolerance. A varroa-tolerant colony, due to its inability to control its mite population, will eventually suffer from the burden of its parasites and pathogens.
Managed apiaries vs. natural evolution
We might expect the hand of evolutionary pressure (natural selection) to come up with a “benign” mite, or a “stable” host-parasite relationship, but that’s not gonna happen so long as honey bees exist as managed livestock. This is because there’s no downside to varroa (or its associated viruses) if they inadvertently kill their host colony — so long as we keep providing fresh colonies to replace them.
In addition, our keeping of hives of bees closer than a mile apart means that mites can use the bees themselves as vectors to transmit those parasites to other hives in the vicinity. As pointed out by Ewald [[iii]], parasites that spread via flying vectors can be transmitted effectively from sick or dying hosts to new hosts, and therefore are not constrained from evolving to become even more virulent. (This would be the case whether we’re talking about the vector being mosquitoes, or drifting or robbing bees.) On the other hand, parasites that require their hosts to remain alive and healthy enough to transmit the parasite vertically to their offspring (in the case of bees, to their swarms), may develop a stable host-parasite relationship (as in the case of humans and our body lice or follicle mites).
Practical application: The above means that there’s no evolutionary pressure upon either varroa or Deformed Wing Virus to become less virulent — The Varroa Problem is not going to get better until we start keeping resistant bees. Thus our only long-term solution is to select for honey bee stocks that are able to reduce varroa’s reproductive success to the extent that it becomes only a minor pest in the colony. Selective breeding is simply human-directed evolution; in this case working with Nature for the benefit of our beloved (and beleaguered) honey bees.
Background
For those who have not been following my “Walking the Walk” series on breeding for varroa resistant bees, you can catch up here [[iv]].
Practical application: My sons and I are running a long-term proof of concept experiment, for the benefit of the commercial queen breeders/producers. My goal is to determine the plausibility/feasibility, labor cost, and rate of progress from a simple “traditional” breeding program, starting with one’s own mongrel stock.
Our experimental program involves managing a dedicated breeding population, by the open-mating (within that breeding population) of a yearly cohort of at least 1000 new queens, all bred from roughly 30 selected breeder queens from the previous year. The selected breeders each spring are chosen from colonies that exhibited the ability to hold their mite infestation rate to near zero over the course of an entire year (without any treatments), but just as importantly, continue to have the desirable properties of being gentle and productive.
I’m intentionally avoiding instrumental insemination, genetic analysis, brood dissection, diligent maternal line tracking, or other costly or time-consuming methods. Our only assessment method is taking mite washes (roughly 2000 per year), coupled with visual inspection for general colony qualities. Any colonies rejected as potential breeders are treated; no colonies need be lost to varroa.
The necessity of Mechanical Agitators
The key to this method is to be able to perform rapid-fire mite washes. This requires portable mechanical agitators, and a system that allows a crew to work together without confusion, described at [[v]]. Since that article, I’ve spent many hundreds (my wife says thousands) of hours in the shop, perfecting new generations of portable mite wash agitators (Figure 1).
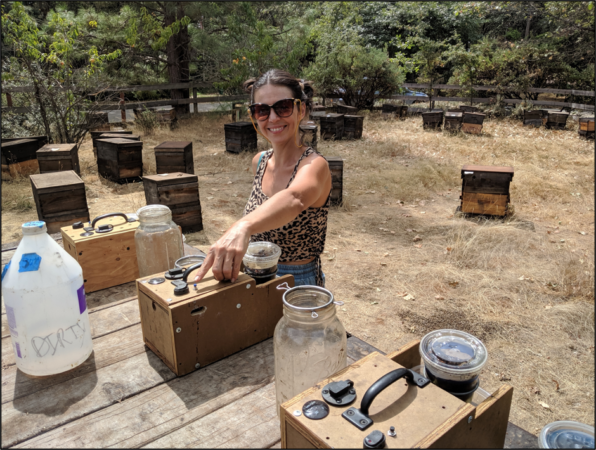
Fig. 1 I built several of these Generation 2 portable mite wash agitators. My assistant Brooke Molina has her finger over the start button. The agitator then performs ~300 revolutions and shuts itself off after 60 seconds. We’ve validated that this agitation recovers of at least 95% of the mites.
The agitators above have served us very well, but when we shifted from using alcohol to Dawn detergent instead, I realized that I could improve them further. After building, testing, and fine-tuning many designs and prototypes, I’ve now developed Generation 4, with better cups, and a simpler design (Figure 2).

Fig. 2 The Gen 4 agitator is specifically designed for use with Dawn detergent, as well as for beekeepers to be able to build their own from off-the-shelf components. This model utilizes a sturdier screw-top jar, and we’ve validated 99% mite recovery in 60 seconds. It includes a pull-out magnifying mirror and holder for rapid counting of mites. I intend to publish the plans in an upcoming article, and may even make up kits for assembly.
Figure 2 shows the agitator sitting on a pull-out table that I built in the back of my Honda CRV, set up for us to rapidly get going when we pull into a yard. With these portable agitators, we can perform mite washes (including opening the hive, taking the sample, closing the hive, agitation and counting, and writing the mite count on top of the hive) at the rate of 3-4 man-minutes per hive (a crew of three of us can easily determine the mite count for every colony in a yard of 48 hives in less than an hour). At $25 per hour, that works out to about a buck and a half labor cost to determine each colony’s infestation rate (that’s less than the cost of some treatments). And we can make an immediate management decision for each colony.
Practical application: Without such portable agitators, performing the number of mite washes necessary for our breeding program wouldn’t be feasible. We find that our savings from not spending money on unneeded mite treatments, as well as the identification of colonies that need more than “the usual” treatment (or that have other issues), more than pays for the cost of labor involved in performing the mite washes.
The resistant colonies
The thing that keeps us going with this demo experiment is the beauty of the colonies that we’ve identified as being mite-resistant — which, if we hadn’t performed mite washes on them, we wouldn’t have known even existed in our operation! (Figure 3).

Fig. 3 The index card on this booming double-deep colony after almonds (with an undisturbed green drone frame), shows the counts for mites in samples of a half cup of bees, taken over the course of a year. (We allowed the mites to build up from the starting nuc in April until the first wash in July.) It’s not unusual for the count to spike in November as the colonies briefly go broodless. Even the count of 7 is only about a 2% infestation rate as the bees go into winter. In the case of this colony, the count expectedly dropped again by the March assessment, after the colony had returned from pollination, at which time most of the mites were back in the brood. For what it’s worth, although it needed no treatments, this colony would not have passed the bar to make it as a breeder.
Of our “potential breeder” pool of resistant colonies each year, to stay in the running, a colony must not only maintain a mite count of near zero for the course of an entire year, but also be gentle, healthy, build up quickly, and be productive as far as honey. That subsequently eliminates a goodly portion of those colonies marked as “potential breeders” at the first assessment.
We take most all of our potential breeders to almond pollination. When I take their mite counts in March, any that are not busting at the seams with bees, or that did not put on plenty of honey in the orchards, are no longer considered as breeders.
Practical application: The remaining breeder pool that we actually graft from (around 30) are the queens of colonies that not only completely controlled varroa, but that any beekeeper would be delighted to have in their own operation, as evidenced by the responses of the several large professional beekeepers who have assisted me in breeder selection (Figure 4).

Fig. 4 This colony laughed at varroa. Brooke said it all one day when she quipped “Zeroes are heroes.” Note the lack of a November spike in count. We didn’t recover a single mite in any of the four samples taken from this colony. If its count was still 1 or less in March, it would be considered as a breeder (at low mite infestation rates, there’s virtually no statistically-significant difference between a count of 1 or 0).
Practical application: The mite counts of this colony are impressive, since a non-resistant untreated colony under our management system will typically reach a mite count of well over 50 by September. Unfortunately, we’ve found that the mite resistance of a colony as a whole does not necessarily predict the performance of daughter colonies. For most of these multiple-zero colonies, daughters bred from their queens only exhibit the breeding population average for resistance. So far we haven’t seen a strong degree of heritability for the trait.
The above frustrating fact may be about to change, since the daughters of a couple of last year’s queens exhibited strong heritability, with up to 50% of their daughter colonies showing resistance. You may have guessed that we’ll be breeding heavily from them this season! In any case, we’re continually impressed by how well our Zero Heroes perform.
PROOF OF CONCEPT: Our mite-resistant colonies are often the strongest and most productive colonies in a yard. Many ask me whether we sacrifice gentleness for mite resistance (Figure 5).
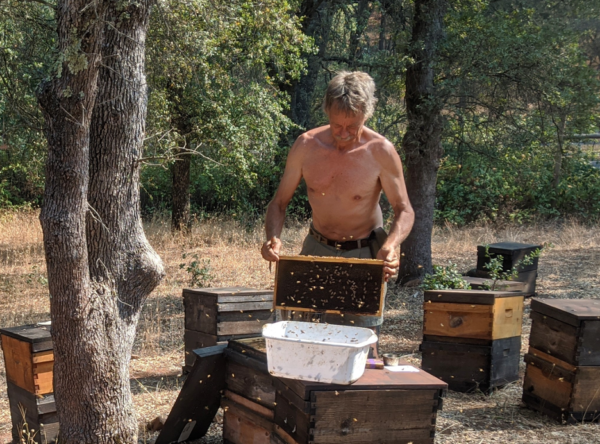
Fig. 5 Eric snapped a shot of me as I performed August mite washes in hot weather. I often knock out 50 mite washes in an afternoon. It’s easy to perform the bioassay for gentleness — if I start getting stung, that colony doesn’t make grade.
So what’s our progress so far?
In a recent project by De la Mora [[i]], working with the Ontario Queen Breeders Association, they appeared to obtain a substantial increase in mite resistance after only two generations, using a selection and breeding method similar to mine. That rate of success is better than that of any other breeding program that I’m aware of, so I hope that their good luck continues! For the rest of us, it’s a much slower slog. My sons and I are going into our sixth year of very strong selection.
I recently realized that we may have been inadvertently kicking a proportion of potentially-resistant colonies out of the program at our first mite wash assessment in June or July, when we go ahead and treat any colony with a count of more than 1 mite (we rejected anything above zero in our first years). At that low of an infestation rate (1 mite per 315 bees equals a 0.317% infestation rate — that’s less than a third of a percent), we can calculate the probability of getting different mite wash counts (Figure 6).
[i] De la Mora A, et al. (2020) Selective breeding for low and high Varroa destructor growth in honey bee (Apis mellifera) colonies: Initial results of two generations. Insects 11(12):864.

Fig. 6 I used the binomial calculator at stattrek.com to work the figures. At an average mite infestation rate of a 1 mite per 315 bees (probability of 0.00317), the chances of getting a wash count of zero is 37%; of seeing exactly 1 mite also 37%; leaving a 26% chance of getting 2 or more mites in a wash. That’s a 26% chance of rejecting a potential breeder right off the bat!
Since our records indicate that roughly half the colonies with mite counts of 1 or 0 in June maintain counts below 7 by the next spring, that means that we’ve likely been excluding (and treating) a lot of what were actually resistant colonies, since we’ve been rejecting a very large number of colonies in June or July that had counts of 2 (Figure 7).
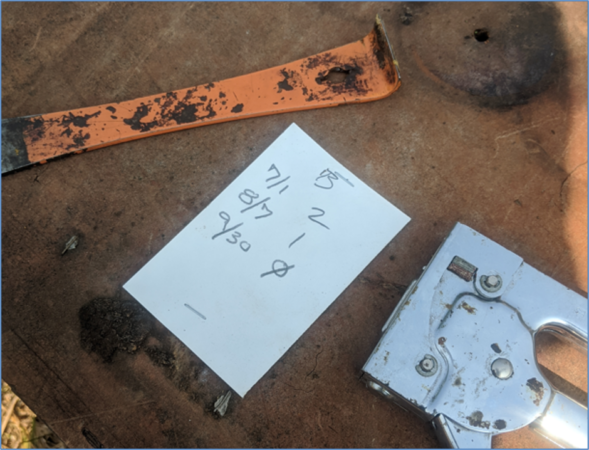
Fig. 7 Out of curiosity, I didn’t treat a few colonies that had mite counts of 2 in early July last year to see where they’d go. The above tag answered that question for this colony! My guess is that we’ve been overlooking a large number of colonies that were actually resistant.
Practical application: It’s a goodly amount of work to perform the first washes on well over 1000 hives in June or July. We’ve applied severe and unsparing selective pressure at the first wash, in order to reduce the number of hives to follow up on. But as with Dr. John Kefuss’ experience, we’ve also observed that resistant colonies are often able to take mite counts back down on their own. So it boils down to how many potential breeders you want to track with additional washes. Luckily, even those resistant but rejected (and thus treated) colonies will still wind up producing drones for next year’s matings.
So where do we stand? Last year was a tough year for our bees. They got hit by severe drought, forest fires, flooding, snow and falling tree damage, bear damage, and then a cold snowstorm just after they started ramping up broodrearing prior to almond pollination. We lose very few colonies to mites, but last year the environment was devastating to our bees. Eric and Ian were barely able to fill our pollination contracts for almonds (although those that went graded well).
That said, of the thousand-plus colonies returning from almonds, we still had 126 tracked (and untreated) potential breeders that exhibited mite wash counts in March of less than 7 (less a 2% infestation rate after an entire year without treatment). Figuring in weather, fire, and bear losses of marked potential breeders, and factoring in our over-culling at our first summer wash, I estimate that between 15% to 20% of our 2021 colonies exhibited strong mite resistance (Figure 8).
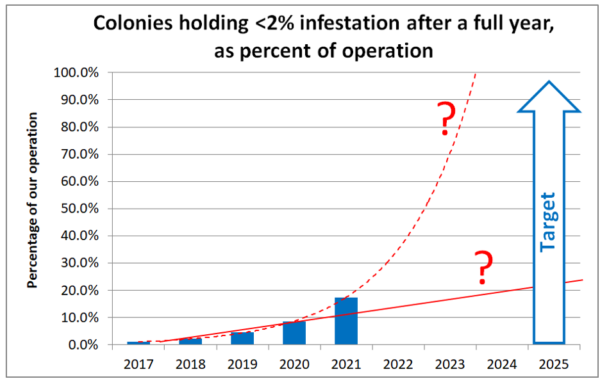
Fig. 8 The above chart reflects my “best guess” proportions of varroa-resistant colonies in our operation over the years. And in one yard this year, we hit 50%. We’ve still got a ways to go until we get to the 95% level at which I might start claiming that our stock is “mite resistant,” but our progress to date is encouraging. Will we slowly make linear progress (the solid red line), or will our results continue to track exponentially (the dashed red line)? I’ll return to this question in my next article.
Practical application: Compared to selective breeding programs to improve the productivity of agricultural crops or animals, which involve changing the morphology, physiology, and energy efficiency of the organisms, to breed for mite resistance, we may only need to flip a couple of behavioral switches. Maybe someone snuck some cannabis leaf into my smoker, but I’m crossing my fingers that our progress will continue to track the dashed line!
A built-in lag inherent in open mating programs
In a breeding program, one can perform either positive selection or negative selection (or a combination of the two). In our own breeding population, in which 99%+ of the colonies would be considered as being “gentle,” it’s easy to practice negative selection against the occasional queen whose colony turns out to be a bit spicy. On the other hand, if we were breeding solely for honey production, we might positively select as breeders only the queens from the few colonies that put on exceptional amounts of honey. In the case of breeding for varroa resistance, we’re doing both — breeding only from the queens whose colonies were exceptionally good at keeping mites in check (positive selection), while simultaneously removing from the breeding population any queens whose colonies appeared to require treatment or didn’t make much honey (negative selection). But unfortunately, there’s a built-in two-year lag for such negative selection, which acts as a drag against the rate of progress of our program.
Understand that the genetics of drones come only from their mothers (whose alleles in turn consist of a mixture of those from their own mothers and fathers). Therefore, since we breed only a single generation of queens each year, the alleles of the drone pool that they will mate with will come from those drones’ grandfathers and grandmothers — reflecting the genetics of the breeding population from two years earlier. Thus our “directed evolution” of the genetics of the drone pool will always lag behind that of the genetics of the breeder queens selected each year. The genetics of the females of the resulting colonies come 50% from the chosen breeder queens of that year, and 50% from the drones whose genetics reflect that of their grandparents. (Yes, go ahead and reread and digest this paragraph.)
Practical application: In a natural breeding population, this genetic conservation of alleles from several generations back helps to prevent dangerous genetic bottlenecking in the case of a sharp reduction in the size of a breeding population of bees due to environmental events such as severe drought or other weather event, landscape-scale fire, or a devastating disease (the stored mixture of spermatozoa in any surviving queen’s spermatheca conserves the local population’s genetic diversity). Unfortunately, this built-in conservation of alleles also slows down the progress of any human-directed selective breeding program that uses open mating, since the alleles of each year’s drone pool reflects the alleles of the population from two years earlier.
Exhibit A: Mite-count tracking for our 2022 breeders
So after taking mite washes after almonds, I needed to select this year’s breeders. I’m guessing that you might like to see how their mite counts tracked over the course of the year (Figure 9).
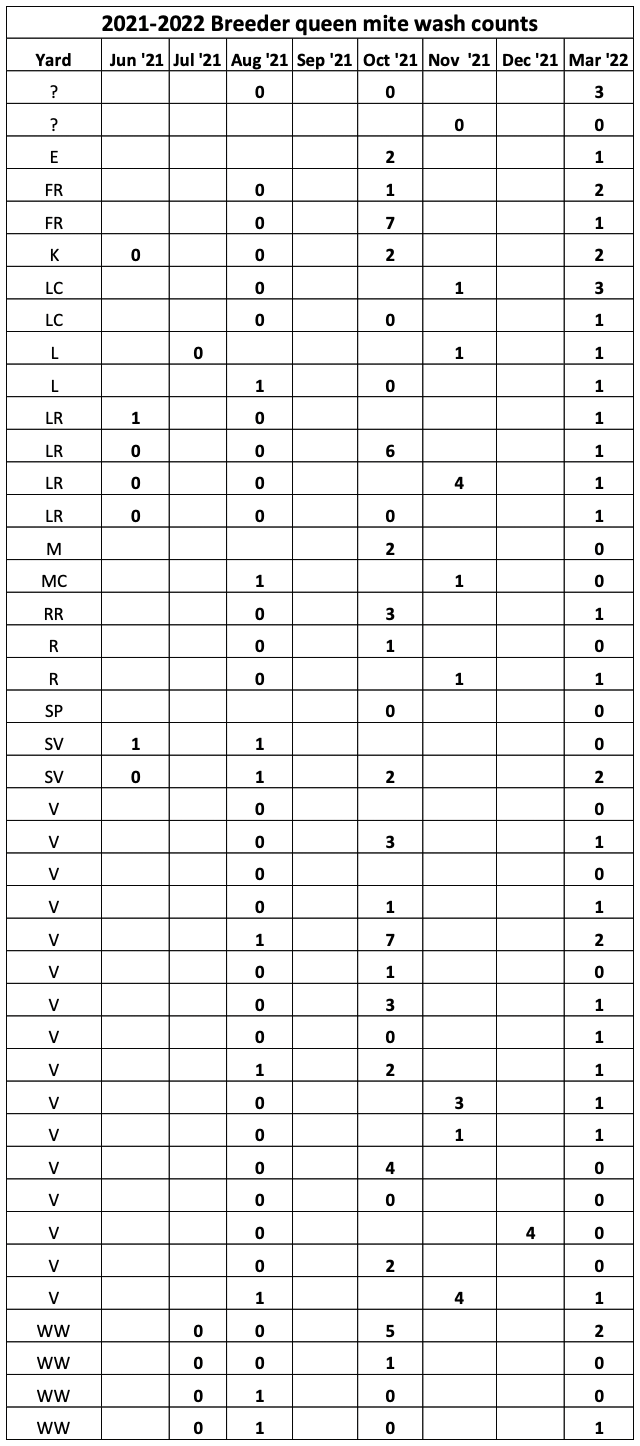
Fig. 9 In 2021, in many of our yards mite counts didn’t get high enough for selection until July or even August, and some didn’t even get checked until November. Note that over a third of this season’s breeders exhibited mite counts of zero after a full year without treatment (their prior count histories indicate that those zero counts in March weren’t flukes).
The yard abbreviations indicate different queen bloodlines, so in order to maintain genetic diversity, I included breeders from a diversity of yards, which required me to include a few with March counts of above 1. We will again requeen our entire operation this year with daughters from these queens, especially those from Yard V (in which 24 of the 48 colonies exhibited strong resistance). I’m especially interested in that bloodline, which I plan to use for some isolated-yard inbreeding later this season.
Coming
Next month I’ll take a dive into our resistant colonies’ mechanism for resistance and the genetics involved.
References
[1] Le Cont, Y, et al. (2020) Geographical distribution and selection of European honey bees resistant to Varroa destructor. Insects 11: 873. doi:10.3390/insects11120873
[2] Ayres, J & D Schneider (2008) Two ways to survive an infection: what resistance and tolerance can teach us about treatments for infectious diseases. Nat Rev Immunol. 8(11): 889–895.
[3] Ewald, P (2004) Evolution of virulence. Infectious Disease Clinics 18(1): 1-15.
[4] 2017 plan: https://scientificbeekeeping.com/the-varroa-problem-part-6a/
2018 update: https://scientificbeekeeping.com/selective-breeding-for-mite-resistance-1000-hives-100-hours/
2019 update: https://scientificbeekeeping.com/selective-breeding-for-mite-resistance-walking-the-walk/
[5] The Varroa Problem: Part 10-Smokin’-Hot Mite Washin’ – Scientific Beekeeping
[6] De la Mora A, et al. (2020) Selective breeding for low and high Varroa destructor growth in honey bee (Apis mellifera) colonies: Initial results of two generations. Insects 11(12):864.